The lunar exosphere, bounded by the emptiness of space and the Moon’s surface, is an atmosphere so thin that atoms rarely collide. This environment, primarily made up of neutral atoms and molecules, is created by the interaction of radiation from the sun, radioactive decay and meteoritic bombardment, balanced by losses into space and recycling back to the surface.
Sputtering by solar H+ and He++ ejects lunar material in the exosphere at low energies; a fraction of which are ionized species. Direct information about the planetary surface composition is carried by sputtered secondary ions and these are observed by a spacecraft ion mass spectrometer. Measurements from the WIND, SELENE, AMPTE, and LADEE missions to the Moon have detected photo-ionized neutrals and secondary ions in the exosphere, including: H2+, He+, C+, Na+, O+, Al+, Si+, K+, CO+, Ar+, Fe+, and Ca+ [Hilchenbach et al. 1993; Stern 1999; Yakota et al. 2009, 2014; Halekas, J. S., et al. 2015].
Relationship Between Solar-Wind Derived Secondary Ions and Lunar Surface Composition
To improve the understanding of the relationship between the lunar surface composition and solar-wind derived secondary ions, as well as guide the design of spacecraft mass spectrometers, secondary ion mass spectra (Figure 1) were obtained during 4 keV He+ irradiation of lunar soils returned by the Apollo missions. For lunar analogs, < 1 mm grains of two mature soil samples, mare 10084 and highland 62231, as well as a Corning glass lunar-simulant and the silicate-mineral olivine were used. X-ray photoelectron spectroscopy (XPS) was used to measure the sample surface compositions, and a Hiden Analytical EQS secondary ion mass spectrometer was used to acquire SIMS spectra.
For this article, positive SIMS spectra were measured at ejected ion energies from 2 to 36 eV, which is considered to be a range where the instrument transmission is expected to be almost constant as a function of energy. Before collecting spectra, the extraction lens voltages were optimized for ions of mass 27 (Al+). The sample surface was charge neutralized during SIMS data collection using low energy (≤ 4 eV) electrons, because the lunar soils charged considerably under ion bombardment.
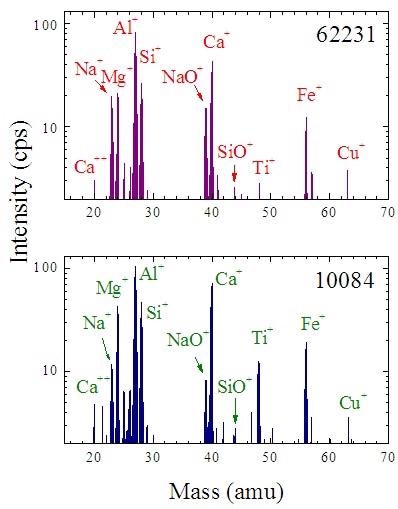
Figure 1. Secondary ion mass spectra of mature, lunar mare soil 10084 and highland soil 62231 for 10 eV ions ejected from the surface by 4 keV He+. Cu+ is an impurity and not intrinsic to the sample.
A one to one correspondence with the surface composition is not shared by sputtered secondary ion intensities, but these intensities are dependent on primary ion type, the local composition of the solid matrix and atom ionization potential. Therefore, it is essential to determine yields on calibration standards of known composition that closely match the target of interest. Species-dependent relative yield factors were established in order to correlate the secondary-ion count rates with the atomic surface composition. It is possible to use the correction factors together with Monte Carlo simulations of solar wind bombardment in order to predict the sputtered ion spectra for planetary satellites, asteroids and other airless bodies [Schaible et al., in prep.].
Additionally, it was observed that SIMS spectra change with fluence, reaching equilibrium only after ~4 x 1017 He+ cm-2 (approximately 9000 years on the lunar surface). The spectra show that ion irradiation preferentially removes species that are weakly bound such as O and Na, enriching the surface in the more refractory materials: Mg, Al, Ti, Ca, and Fe. Secondary ion energy spectra were also collected for selected masses, shown in Figure 2. It is seen that the distributions all rise rapidly, peak below 15 eV, and then drop off slowly with energy, typical of insulating surfaces.
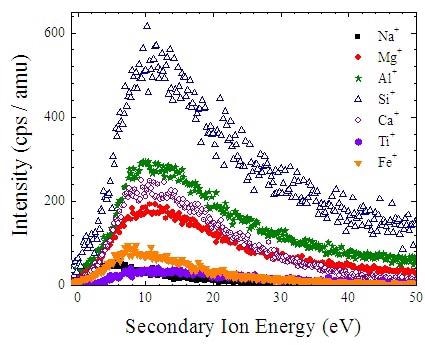
Figure 2. Energy spectra of secondary ion ejected from mature lunar mare soil 10084 by 4 keV He+.
Conclusion
This article demonstrates the possibility of measuring appreciable numbers of secondary ions ejected by solar wind type ions from lunar soils within minutes in the laboratory. These measurements can help guide the exploration of the Moon and understand the surface composition of other airless planetary bodies exposed to magnetospheric, solar-wind or ionospheric ions.
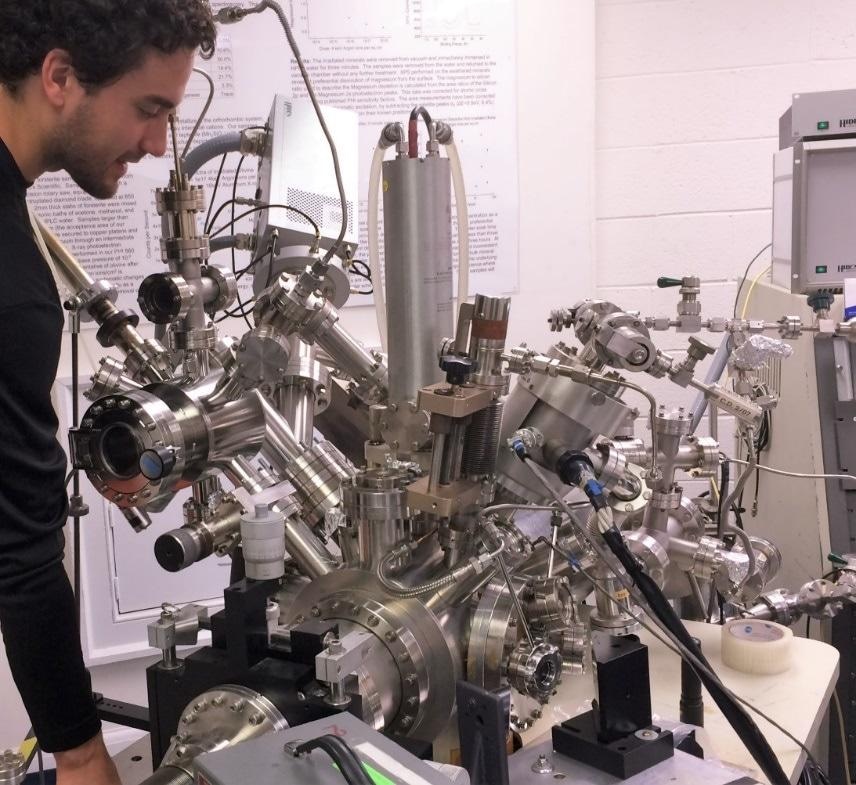
Figure 3. Experimental setup with Hiden Analytical EQS secondary ion mass spectrometer on a modified PHI 560 XPS/SAM system
Project summary by:
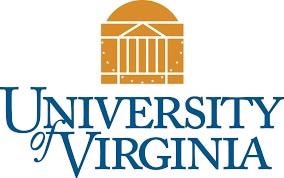
C. A. Dukes and M. J. Schaible
University of Virginia
Laboratory for Atomic and Surface Physics,
Charlottesville, VA 22904
USA
Paper Reference
C. A. Dukes & R. A. Baragiola (2015) “The lunar surface-exosphere connection: Measurement of secondary-ions from Apollo soils” Icarus 255, 51-57
Bibliography
Halekas, J. S., M. Benna, P. R. Mahaffy, R. C. Elphic, A. R. Poppe, and G. T. Delory (2015), Detections of lunar exospheric ions by the LADEE neutral mass spectrometer, Geophys. Res. Lett., 42, 5162–5169.
Hilchenbach, M., Hovestadt, D., Klecker, B., and Mӧebius, E. (1993) Observation of energetic lunar pick-up ions near earth, Adv. Space Res. 13, (10) 321–324.
M.J. Schaible, C.A. Dukes, R.E. Johnson, A.C. Hutcherson, P. Lee, M.R. Collier, (In Prep.) Solar wind sputtering rates of small bodies and ion mass spectrometry detection of secondary ions, Journal of Geophysical Research
Stern, A (1999) The lunar atmosphere: history, status, current problems, and context, Reviews of Geophysics, 37, 4, 453–491.
Yokota, S., Saito, Y., Asamura, K., Tanaka, T., Nishino, M.N., Tsunakawa, H., Shibuya, H., Matsushima, M., Shimizu, H., Takahashi, F., Fujimoto, M., Mukai, T., and Terasawa,. T., (2009) First direct detection of ions originating from the Moon by MAP-PACE IMA onboard SELENE (KAGUYA), Geophys. Res. Lett., 36, L11201.
Yakota S., Tanaka, T., Saito, Y., Asamura, K., Nishino, M.N., Fujimoto, M., Tsunakawa, H., Shibuya, H., Matsushima, M., Shimizu, H., and Takahashi, F. (2014) Structure of the ionized lunar sodium and potassium exosphere: Dawn-dusk asymmetry, J. Geophys. Res. - Planets, 119, 798–809.
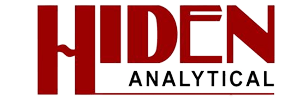
This information has been sourced, reviewed and adapted from materials provided by Hiden Analytical.
For more information on this source, please visit Hiden Analytical.