Introduction
In recent years, it has been widely demonstrated that the interaction between ultraviolet radiation and a semiconductor catalyst has a strong potential for the destruction of toxic organics in industrial wastewater [1, 2, 3]. Photocatalysis has many potential applications for treating water containing organics, for removing metals from water, and for splitting water. All these processes are interrelated. Band gap illumination of a semiconductor particle suspended in water causes electronic transitions from valence band to conduction band creating electron-hole pairs. Simultaneously, in the presence of a fluid phase, adsorption occurs and an electron transfer proceeds towards acceptor molecules (oxidizing species), whereas positive holes are transferred to donor molecules [4].
A major center of attention of current catalysis research is the elucidation of critical structure-function relationships for existing solid catalysts that can be subsequently utilized in the design of materials. These studies necessarily require the combination of materials synthesis, characterization and catalytic testing.
Inoue et al., have shown that sodium hexatitanate Na2Ti6O13, combined with an active phase such as Ru, has the capability of invoking a photocatalytic decomposition of water to produce H2 and O2 at approximately the stoichometric ratio [5]. This mixed oxide is one of the alkali-metal titanates belonging to a chemical formula of M2TinO2n+1 (alkali-metal atom).
On the other hand, previous studies about the ternary phases formation in the system BaO-Li2O-TiO2 for compositions containing > 50% of TiO2, demonstrated that the phase A, centered in Ba3Li2Ti8O20, showed electronic conductivity [6, 7], which indicates that this compound can be used as semiconductor. The crystal structure of Ba3Li2Ti8O20 is analogous to that of Na2Ti6O13 and Ba2Ti6O13 [monoclinic, space group C2/m (no.12)] in which edge-sharing trimers formed by three kinds of octahedra: the Ti(1)O6, Ti/Li(2)O6 and Ti(3)O6, share corners with other trimers forming infinite ribbons with a zig zag arrangement. The Ba atoms are inside the tunnels which were formed between the ribbons of edge-sharing octahedra [8].
We assume that if the structure of the catalyst is analogous, the ternary titanate Ba3Li2Ti8O20 or other titanates with the same structure, should exhibit also photocatalytic behavior. To probe this assumption we carried out the decomposition reaction of 2,4 dinitroaniline over the solids obtained under the same degradation conditions. However, in photocatalysis the catalytic activity of a semiconductor is not only determined by electronic properties, but also by particle size, surface area and its crystallinity.
The chemical history and thermal treatment of the sample is very important in the activity of a semiconductor [9-14]; hence, in this work, we have prepared the ceramic compounds Li2Ti6O13, Na2Ti6O13 and K2Ti6O13 by sol-gel method and by solid state reaction in order to study their photocatalytic properties and the influence of the preparation method. The Ba3Li2Ti8O20 was only prepared by sol-gel technique in acid and alkaline conditions.
Experimental
Synthesis
For AmM2nO4n+1 (A= Li, Na, K; m= 2, n= 3) sol-gel synthesis, we use as metallic precursors titanium n-butoxide (98+%, Strem Chem) and the corresponding alkali-metal acetate (99.5%, Aldrich), t-butanol (99.5%, J. T. Baker) was used as solvent and glacial acetic acid (Aldrich 99%) was added under continuous stirring to reach pH 3.
For Ba3Li2Ti8O20 sol-gel, barium isopropoxide (Aldrich Chemicals 99.9%), lithium acetate dihydrate (Sigma 63.4%) and titanium isopropoxide (Aldrich Chemicals 97%) were the metallic precursors. Ethanol (99.9% PROQUIM) was used as solvent and glacial acetic acid (PROQUIM 99.8%) was added under continuous stirring to reach pH 3 [15]. The reaction mixture was heated by reflux at 70°C. The gels obtained were dried in air at 80°C for 48 h (fresh sample) and different portions were heated at 200, 400, 600 and 800°C for 6 h. These ternary compounds were also prepared at pH 9 by sol-gel and ammonia hydroxide was used instead of acetic acid [16].
Only the binary compounds were synthesized by solid state reaction. An intimate mixture of TiO2 and the corresponding carbonates (Aldrich, 99%) was heated at 1250ºC over a period of 48-72 h.
Characterization
Infrared absorption spectroscopy was done in a Perkin Elmer Fourier transform infrared spectroscopy (FT-IR) Spectrometer Paragon 1000 PC in order to analyze the hydroxyl groups on the catalysts. Nitrogen adsorption isotherms of the samples were measured by a gas sorption instrument (Micromeritics ASAP 2000). The specific surface area was calculated by the BET equation. The samples were analyzed by X-Ray Diffraction (XRD) technique in a Siemens D-5000 diffractometer with Cu Kα radiation ( λ = 1.5418Å ). The diffuse reflectance spectra of the solids were obtained with a Perkin Elmer spectrophotometer Lambda 12 coupled to an integration Labsphere RSA-PE-20. A Spectralon standard USRS-99010 with 100% reflectance was used as a reference.
Photocatalytic Activity
Dinitroaniline Decomposition
Photocatalytic experiments were carried out at room temperature, 200 mg of each catalyst was added to a flask containing 500 ml of an aqueous solution of 2,4-dinitroaniline at 30 ppm. Under stirring, the solution was irradiated in a closed box with a UV lamp Black-Ray model XX-15, which emits radiation at λ=254 nm at an intensity of 1100 μW/cm2. The reaction rate was followed by taking aliquots every 10 min and then analyzed in an UV-Vis spectrophotometer Perkin-Elmer Lambda-12. The concentration of 2,4-dinitroaniline was calculated from the absorption band at 346 nm present in the UV-Vis spectra of this substance. For a data evaluation we applied the Langmuir-Hinshelwood model, using the equation for heterogeneous photocatalytic reactions in a batch reactor [17].
To estimate the time in which C = Co/2, we use:
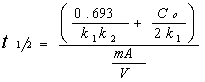
Where V is the liquid volume, C the substrate concentration, t the time, m the mass of catalyst, A the adsorption sites by g/cat, k1 the apparent rate constant and k2 the apparent adsorption constant.
Chromium VI reduction
The crystalline forms of Na2Ti6O13 and Ba3Li2Ti8O20 and the ceramic compounds Na2ZrTi5O13 and Na1.8Ba0.3Ti5.9O13 prepared by sol-gel, were used as catalysts in the photacatalytic reduction of Cr(VI) to Cr(III). In these experiments 100-150 mg of each catalyst were added to a flask containing 200 ml of an aqueous solution of K2Cr2O7 (reagent grade) at 30 ppm. Initial pH of the solution was 1-2. The liquid was maintained under continuous stirring while it was irradiated in the closed box with the same UV lamp used in 2,4 dinitroaniline degradation. Concentration of Cr(VI) was analyzed by UV spectroscopy using diphenylcarbazide [18].
For all experiments, the starting solution with the catalyst was allowed to equilibrate in the absence of UV illumination for 30 min.
Results and Discussion
X Ray Diffraction
Figure 1 shows XRD patterns of the xerogel evolution of lithium titanate with temperature. When the powder is heated at 200 and 400°C a few peaks are observed, which is indicative of a structure almost amorphous. However, a small peak is observed at 25° associated to the main peak of TiO2 anatase phase. When fresh sample is heated at 800°C, the xerogel is transformed to yield a crystalline material with a powder pattern similar to that reported for Li2Ti6O13. By solid state method, according to XRD studies, it was not possible to obtain this compound, which is due to some loss of lithium by volatilization at higher temperatures.
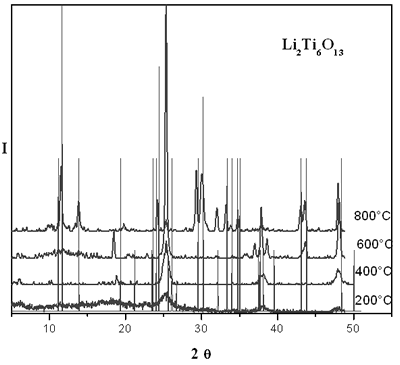
|
Figure 1. XRD patterns of thermal evolution of sol-gel lithium titanate.
|
The formation of Na2Ti6O13 compound was achieved when the sample obtained by sol-gel was heated also at 800°C. At 600ºC the anatase form is present. By means of ceramic method, this compound was formed at 1250°C for 48 h.
The XRD patterns of thermal evolution of xerogel potasium titanate are shown in Figure 2. We can see that the most important reflections for the compound K2Ti6O13 were fitted at 600°C. The small reflections point out the presence of potassium hexatitanate formed with a particle size in the nanocrystal interval. It is evident in the sample heated at 800°C that the reflections observed do not correspond to the K2Ti6O13 structure. This difractogram is similar to that reported for TiO2 rutile form which is stable at higher temperatures, it is possible that this sol-gel phase is metastable and up to 600ºC it is becoming into other crystalline specie, then the majority phase in the powder at 800°C is rutile. By solid state method the K2Ti6O13 compound was obtained at 1250°C by 72 h.
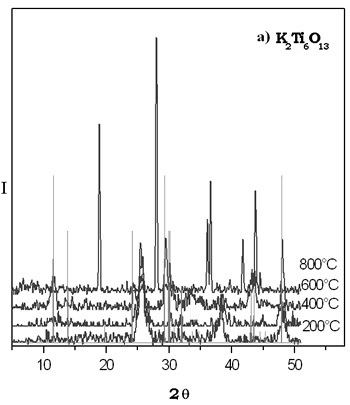
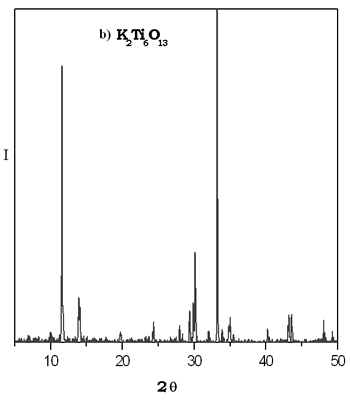
|
Figure 2. a) XRD patterns of thermal evolution of potassium titanate gel. b) XRD pattern of K2Ti6O13 prepared by solid state reaction.
|
The formation of Ba3Li2Ti8O20 sol-gel was achieved at pH 3 when a fresh sample was heated at 800°C. The crystalline structure was confirmed by the XRD pattern similar to that reported by Dussarrat and et al. [8].
When a ternary compound was synthesized by sol gel at pH 9, Rietveld studies demonstrated that Ba3Li2Ti8O20 is formed with a particle size in the nanocrystal interval at 600°C [19]. Nanocrystallinity of the compound is initiated at lower temperature when the sol-gel method is performed at alkaline pH. The sample annealed at 800°C showed a XRD pattern which corresponds to that reported in the literature to Ba3Li2Ti8O20 structure, but it has higher crystallinity.
Photocatalytic activity
Dinitroaniline Decomposition
Figure 3 shows the reduction of 2,4 dinitroaniline concentration with reaction time on sodium titanate catalyst prepared by sol-gel. Similar graphs were done with the other solids prepared. We can see that the more active sample is the solid obtained at 600ºC when the Na2Ti6O13 structure was formed.
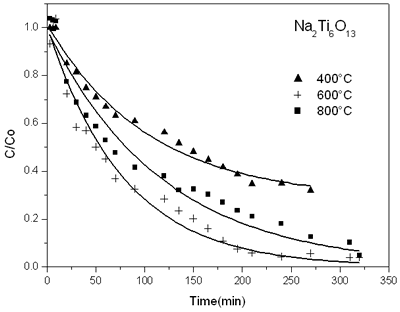
|
Figure 3. Evolution of time function for decomposition of 2,4 dinitroaniline on sodium titanate catalysts sol-gel annealed at different temperatures.
|
The values of k1, k2, k1k2 (apparent reactivity) and t1/2 (apparent half-life) for each catalyst, on the decomposition of the 2,4-dinitroaniline, were calculated applying the Langmuir-Hinshelwood model. These data were estimated from a graph of (Ln(Co/C))/(Co-C) against t/(Co-C).
The photocatalytic activity of each semiconductor catalyst was, therefore, compared by use of the half life time values shown in Table 1.
Table 1. Half life time values of the decomposition of 2.4-dinitroaniline on AmM2nO4n+1 Sol-gel catalysts.
|
Li2Ti6O13
|
400
|
3.13
|
93.75
|
34
|
Li2Ti6O13
|
600
|
3.19
|
17.59
|
57
|
Li2Ti6O13
|
800
|
3.39
|
0.19
|
25
|
Na2Ti6O13
|
400
|
3.15
|
73.1
|
297
|
Na2Ti6O13
|
600
|
3.17
|
60.7
|
67
|
Na2Ti6O13
|
800
|
3.18
|
7.69
|
120
|
K2Ti6O13
|
400
|
3.14
|
----
|
131
|
K2Ti6O13
|
600
|
3.19
|
37.9
|
91
|
K2Ti6O13*
|
800
|
3.46
|
0.51
|
103
|
* TiO2 as rutile form is the majority crystalline phase present
With sol-gel catalysts, we can observe, for lithium samples, that the most active catalyst is the material heated at 800ºC when the structure of lithium hexatitanate is already formed. In the case of sodium sol-gel samples, the best activity was exhibited by the solid heated at 600ºC when the TiO2 anatase form is present. Previous works in the TiO2 sol-gel synthesis [20] showed that at pH3 conditions, anatase is present with small percentages of rutile when the samples are annealed at lower temperatures (200-600ºC). Some investigations on photocatalytic degradation of different organic compounds in water, showed that the anatase form is more active than the rutile phase [21, 22]. In this case the structure is formed from anatase to Na2Ti6O13 at 800ºC.
For potassium materials, the activity is improved with the catalyst heated at 600ºC. We have seen that at this temperature the K2Ti6O13 structure is achieved with a small particle size which favored the photocatalytic activity. At 800ºC, the rutile form is present and several works have reported a lower activity for this catalyst. The rate of the hole and electron recombination is higher for rutile than for anatase due to the semiconducting properties of two TiO2 phases that are slightly different [23].
Among all sol-gel AmM2nO4n+1 catalysts probed we can see that the best activity was achieved with lithium hexatitanate obtained at 800ºC. The TiO6 octahedra distortion is caused by the alkaline atom size. For these experiments the less distorted structure exhibit the major activity on the photocatalytic degradation of 2,4-dinitroaniline.
On the other hand, according with half life time calculated with the catalyst prepared by ceramic method (Table 2), we can appreciate a great catalytic activity similar to lithium hexatitanate sol-gel. In these materials the crystallinity is higher and the band gap energy values calculated from its UV-vis spectra were 3.04 and 3.08 eV for Na2Ti6O13 and K2Ti6O13 respectively, whereas for the same compounds obtained by sol-gel this values were 3.4 eV for Li2Ti6O13 and Na2Ti6O13 ; nevertheless for K2Ti6O13 was 3.1eV.
Table 2. Half life time values of the decomposition of 2.4-dinitroaniline on AmM2nO4n+1 catalysts prepared by solid state reaction.
|
Na2Ti6O13
|
1250
|
3.08
|
0.4
|
21
|
K2Ti6O13
|
1250
|
3.04
|
0.4
|
16
|
Once Ba3Li2Ti8O20 was used as a photocatalyst, we found a higher photocatalytic activity expressed as t1/2 (Table 3), for the solid obtained at pH 3 and annealed at 800°C (t1/2= 26 min). The adsorption capacity of this catalyst is favored due to its higher degree hydroxylation, hence the OH groups behave as adsorption centres. At this temperature (800°C), the crystalline structure of Ba3Li2Ti8O20 is formed according to XRD studies.
Table 3. Half life time values of the decomposition of 2.4-dinitroaniline on Ba3Li2Ti8O20 sol-gel catalysts.
|
3
|
400
|
3.09
|
66.51
|
44
|
3
|
600
|
3.14
|
38.08
|
33
|
3
|
800
|
3.18
|
6.17
|
26
|
|
|
|
|
|
9
|
400
|
3.48
|
117.83
|
54
|
9
|
600
|
3.44
|
49.30
|
24
|
9
|
800
|
3.37
|
9.37
|
28
|
For the ternary catalyst synthesized at pH9 the photodegradation is improved with the samples fired at 600 and 800ºC. At basic conditions the sample calcined at 800ºC has the same crystalline structure than under acidic conditions, however, it lost almost all hydroxy groups causing high defects degree yielding vacancies. Defects in an oxide semiconductor are linked to electronic properties. According to the k1 value calculated, in this sample, electronic transference is favoured and it shows a good activity on 2,4-dinitroaniline decomposition (t1/2 = 28 min). However, the adsorption capacity is low due to material sintering that takes place by thermal treatment. With the sample heated at 600ºC obtained at alkaline pH, the activity expressed as t1/2=24min, is closer to those calculated for the samples annealed at 800ºC. This behaviour is due to major specific surface area and the nanocrynstallinity of the ternary compound.
Chromium VI Reduction
Table 4 shows the results of the initial studies on photocatalytic activity of Na2Ti6O13 and Ba3Li2Ti8O20 compared with others isostructural compounds (Na2ZrTi5O13 and Na1.8Ba0.3Ti5.9O13) on the hexavalent chromium photoreduction. The activity was evaluated calculating the fraction of Cr(VI) reduced to Cr(III) after 3.5 h of UV illumination. Kinetics studies are being done in our research group in order to evaluate the reaction rates with these compounds.
Table 4. Cr (VI) photoreduction percent of isostructural materials with monoclinic structure with space group C2/m.
|
Na2Ti6O13
|
86
|
15.13
|
3.74
|
9.16
|
99.30
|
Ba3Li2Ti8O20
|
71
|
15.17
|
3.90
|
9.11
|
98.64
|
Na2ZrTi5O13
|
53
|
-
|
-
|
-
|
-
|
Na1.8Ba0.3Ti5.9O13
|
31
|
15.18
|
3.78
|
9.14
|
98.70
|
Conclusions
When the sol-gel method was used to prepare ceramic powders, the acid conditions caused the formation of the compound with less surface area and less porosity, giving as a result lower catalytic activity. The Eg values depend on both synthesis parameters: pH and thermal treatment. However, according to these results, the photocatalytic activity is favored when the structure of each compound has been formed. The best activity in 2,4-dinitroaniline decomposition, was performed by lithium hexatitanate sol-gel when the crystal structure was formed and also, by Na2Ti6O13 and K2Ti6O13 prepared by solid state reaction, therefore, it seems to be that the crystal structure is an important factor that has to be considered in the photocatalytic process. In these isostructural compounds the atoms arrangement is similar but TiO6 octahedra distortion caused by the alkaline atom size is different. For these experiments the less distorted structure exhibits the major activity on the photocatalytic process. On preliminary studies of chromium reduction, Na2Ti6O13 sol-gel was the best catalyst.
Additionally, the synthesis method plays an important role determining the surface characteristics of the photocatalytic material. It was found that the synthesis of the solid obtained by sol-gel technique under basic conditions improved the catalytic behavior of the material. The sol-gel technique cause the structure formation with different kind of defects formed due to dehydroxylation process during thermal treatment. The method and synthesis conditions cause different number, type and strength of surface active sites which determine the catalytic properties of the catalysts.
Acknowledgements
We acknowledged the financial support provided by Conact through grant 35415-U. We are grateful to Dr. Tessy Lopez for her valuable supervision in sol-gel technique. We also thank Brenda Fernandez and Lucia Castillo for performing the preliminary Cr(VI) photoreduction experiments. J.C. Luna-Urzua wishes to thank the CONACT for awarding of the scholarship.
References
1. S. Chiron, A. Fernández-Alba, A. Rodríguez and E. García-Calvo, “Pesticide Chemical Oxidation: State-Of-The-Art.”, Wat. Res., 34 (2000) 366-377.
2. A. Fujishima, T. N. Rao and D. A. Tryk, “Titanium Dioxide Photocatalysis”, J. Photochemistry & Photobiology C: Photochemistry Reviews, 1 (2000) 1-21.
3. J. P. Wilcoxon, “Catalytic Photooxidation of Pentachlorophenol Using Semiconductor Nanoclusters”, J. Phys. Chem. B, 104 (2000) 7334-7343.
4. J. M. Herrmann, “Heterogeneous Photocatalysis: fundamentals applications to the removal of various types of aqueous pollutants”, Catalysis Today, 53 (1999) 115-129.
5. Y. Inoue., T. Kubokawa and K. Sato, “Photocatalytic Activity of Alkali-Metal Titanates Combined With Ru in the Decomposition of Water”, J. Phys. Chem., 95 (1991) 4059-4063.
6. C. Suckut, R. A. Howie, A. R. West and L. M. Torres-Martìnez, “Synthesis and Properties of Hollandite-Like Ba3xLi(2x+4y)Ti(8-2x-Y)O16”, J. Mater. Chem., 2 (1992) 993-996.
7. L. M. Torres-Martìnez, C. Suckut, R. Jimenez and A. R. West, “Phase Formation and Electrical Properties in the System BaO-Li2O-TiO2”, J. Mater. Chem., 4 (1994) 5-8.
8. C. Dusarrat, R. A. Howie, C. Mather, L. M.Torres-Martìnez and A. R. West, “Crystal Structure of Ba2Li2/3Ti16/3O13“, J. Mater. Chem., 7, (1997) 2103-2106.
9. Ch. K. Chan, J. F. Porter, Y. Guang Li, W. Guo and Chi-Ming Chan, “ Effects of Calcinations on the Microstructures and Photocatalytic Properties of Nanosized Titanium Dioxide Powders Prepared by Vapour Hydrolysis”, J. Am. Ceram. Soc., 82 (1999) 566-573.
10. A. Sclafani, L. Palmisano and M. Schiavello, “Influence of the Preparation Methods of TiO2 on the Photocatalytic Degradation of Phenol in Aqueous Dispersion”. J. Phys. Chem., 94 (1990) 829-832.
11. K. Young and Ching-BinHsieh, “Photocatalytic Decomposition of 2,4-Dichlorophenol in Aqueous TiO2 Suspensions”, Water Research, 26 (1992) 1451-1456.
12. J. Chen, D. F Ollis., W. H. Rulkens and H. Bruning, “Photocatalyzed Oxidation of Alcohols and Organochlorides in the Presence of Native TiO2 and Metallized TiO2 Suspensions (Part I) Photocatalytic Activity and pH Influence”, Wat. Res., 33, [3] (1999) 661-668.
13. J. Chen, D. F. Ollis, W. H. Rulkens and H. Bruning, “Photocatalyzed Oxidation of Alcohols and Organochlorides in the Presence of Native TiO2 and Metallized TiO2 Suspensions (Part II) Photocatalytic Mechanisms”, Wat. Res., 33, [3] (1999) 669-675.
14. C. J. Brinker, R.Sehgal, N. K. Raman, S. S. Prakash and L. Delattre, “Sol-gel Strategies for Controlled Porosity Ceramic Materials: Thin Film and Bulk”, Mat.Res. Soc. Symp. Proc., 368 (1995) 329-334.
15. X. Bokhimi, O. Novaro, T. Lopez, E. Sánchez and R. Gomez, J. Mater. Res., 10 (1995) 2788.
16. Z. Ding, G. Q. Lu and P. F. Greenfield, “Role of the Crystallite Phase of TiO2 in Heterogeneous Photocatalysis for Phenol Oxidation In Water”, J. Phys. Chem. B, 104 (2000) 4815.
17. V. Augugliaro, L. Palmisano, A. Sclafani, C. Minero and E. Pellizeti, “Toxicological and Environment Chemistry”, 16, Science Publishers, Inc., Great Britain, (1988) 89-109.
18. J. Sun, L. Gao and Q. Zhang, “Synthesizing and Comparing the Photocatalytic Properties of High Surface Area Rutile and Anatase Titania Nanoparticles”, J. of the American Ceramic Society, 86 [10] (2003) 1677-1682.
Contact Details
|