Introduction Liquid crystals (LCs) can be easily oriented by contact with surfaces on a macroscopic scale [1]. The phenomenon of orientation of LCs by surfaces is a topic of crucial significance for applications of these materials, especially for liquid crystal displays (LCDs) [2]. To fabricate a commercial product of LCD, uniform orientation of the LC molecules is required. The alignment of a LC by the surface treatment of a substrate has been one of the essential aspects of LC behavior. It is usually achieved by using polymer alignment films deposited on glass substrates. Hence, alignment films are key components in LCDs. Homogeneous alignment occurs when the LC director aligns parallel to the substrate, whereas perpendicular alignment is referred to as homeotropic. To achieve a homogeneous alignment, a preferred orientation to the alignment by physically or chemically treating the surface must be provided. Rubbing the surface has been a simple way of achieving a preferred orientation. However, rubbing may produce dust, static charging and mechanical damage which deteriorates the production yield. One of the more attractive alternatives to rubbing is the generation of a surface anisotropy of an alignment film by photochemical reaction called “photoinduced alignment” [3]. In general, photoinduced alignment is achieved by exposing with both unpolarized and polarized ultraviolet (UV) light on a photoalignment polymer film. There are three main kinds of materials that are used for photoalignment layers. They can be categorized according to the photochemical reaction responsible for the photoalignment, viz. (i) azo–containing polymers (photoalignment by reversible cis–trans isomerization), (ii) crosslinkable materials (photoalignment by photo–dimerization), and (iii) polyimides (photoalignment by photodegradation) [4]. The understanding of surface effects and anchoring in LCs requires profound knowledge of the LC–surface interaction. The nature of the LC–surface interaction is complex and the most important factors include van der Waals interactions, dipolar interactions, steric interactions, hydrogen and chemical bonding, and surface topography [5]. Which interaction plays a major role depends on the prevailing circumstances. Except for the surface topographical part, all contributions are primarily governed by intermolecular interactions between substrate and LCs. Despite the enormous theoretical and practical relevance of surface anchoring, the underlying mechanisms are not very well understood [2]. In particular, there are two models of alignment mechanisms to elucidate surface–induced bulk alignment in the case of nematic LCs, viz. (i) Berreman’s groove model and (ii) molecular epitaxy-like model [6, 7]. The first model is anisotropic LC orientational elasticity in connection with rubbing–induced microgrooves [8-10]. The second model is short–range interactions on the molecular scale [7, 11-15]. In regard to the first model, Berreman proposed that the director field adapts itself to the geometrical topology of the substrate surface to minimize the elastic strain energy arising from the distortions of the director field. The second model is based on interactions between the LC molecules and the substrate. These interactions affect the orientational order of the LC molecules in the vicinity of the substrate; resultant LC molecular order in turn propagates toward the bulk via the intermolecular interactions which tend to align the molecules parallel to each other. The aligning action evolves from the substrate to the bulk LC via a specific interfacial region [2, 16, 17]. This means that the bulk molecules exhibit epitaxial growth of nematic phase from the alignment surface [7, 18]. The anisotropic surface–LC interaction by which the surface governs the orientation of the bulk is often termed surface orientational anchoring [2]. For a better understanding of the surface effect on the alignment of LCs, many studies are paying special attention to the interfacial properties of LCs. To clearly characterize the microscopic features of surfaces and interfaces, many types of imaging techniques have been developed over the years. However, most of their abilities have some limitations. Recently, several laser techniques have been developed for surface probing. Among these, optical second–harmonic generation (SHG) and vibrational spectroscopy by sum–frequency generation (SFG) have been proven to be effective tools for probing orientation and arrangement of surface molecular alignment [6, 19-21]. In SHG technique, the signal increases with decreasing spot size for a given laser power but may be eventually limited by laser–induced surface damage. The problems involved in SHG technique are considerations about the size of the area to be probed, and the surface damage threshold of the material caused by laser heating [22]. Moreover, in regard to SHG technique the probed area should be uniform because inhomogeneous surface area can generate variations in the SHG signal [23]. In this study, we have carried out the investigation by means of a novel surface profiler instrument to visualize a microscopic image of the monolayer arrangement of LC molecules in contact with the surface of a photo–treated PI–VA film. As will be discussed later, the fundamental concept of this surface profiler is based on the detection of angular deflection of a fast–scanning laser beam in a non–contact manner [24]. Laser–induced surface damage effect and limitation on the size of the probed area that are encountered in SHG technique can be overcome because the scan speed of the laser beam spot on substrate surface is 5 mm/ms over a wide range of scanning area (~ 40 mm). In our previous study, we used polyvinylcinnamate as a photoalignment layer [25]. In this work, we used PI–VA as a photoalignment layer that originally exhibited homeotropic alignment. The optical anisotropy of the PI–VA is due to the photodegradation effect under the irradiation of polarized or unpolarized UV light. To elucidate a basic concept of photodegradation effect, we would like to review original works of literature. Hasegawa and Taira [26] first reported that a PI film exposed to linearly polarized ultraviolet (LPUV) light induces a uniform parallel (homogeneous) alignment of LC molecules. The average molecular orientation of the LC molecules is parallel to the film surface and perpendicular to the polarization direction of the LPUV light. They explained this LC alignment from the viewpoint of anisotropic photodepolymerization of the PI main chains. The PI chain oriented parallel to the polarization direction of the irradiated LPUV light is broken more easily than that oriented perpendicular to it. Thus, the orientational distribution of the PI chains becomes anisotropic after LPUV exposure. This anisotropic orientation of the PI chains aligns the LC molecules perpendicular to the polarization direction which corresponds to the direction of the maximum density of undegraded polyimide chains on exposure. Yoshida et al. [27], Li et al. [28], and Iimura et al. [29] also realized that the tilted homeotropic alignment was achieved by irradiation of unpolarized and polarized UV light on a PI film that originally exhibited homeotropic alignment. In these studies, they confirmed that the optical anisotropy of the PI resulted from the photodepolymerization of the alkyl branch in the film. Yoshida et al. [27], and Iimura et al. [29] also demonstrated that the p–component of the incident UV light contributes to the pretilt angle generation but the s–component cannot give a tilted polymer distribution due to the circular symmetry in the plane of incidence. The purpose of this investigation is to study the surface alignment of LC multilayers evaporated on a photoinduced PI–VA film and to visualize a microscopic image of the monolayer arrangement of LC molecules in contact with the surface of the PI–VA film. We aim to investigate the alignment of LC molecules on a periodically patterned substrate surface to see a clear picture of different alignment mechanisms. In this study, the surface alignment of LC multilayers evaporated on a photoinduced PI–VA film was investigated by means of the 3-D surface profiler and the optical polarizing microscope. Concept of the 3-D Surface Profiler Figure 1(a) depicts a fundamental concept of the profile measurement, a curved surface S and a light source L keeping a distance l between them. The scanning direction is along the x–axis as shown in Figure 1(a). The surface profile of the measured object along the x–axis can be expressed by the function f(x). Let us assume that the light beam from the laser light source L is emitted on the curved object at the point P. When the angle θ with respect to the x–axis is much less than 1, the gradient at P can be expressed by: (1) 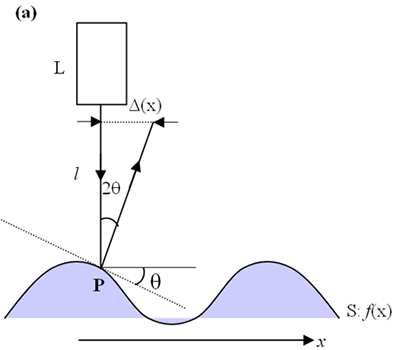 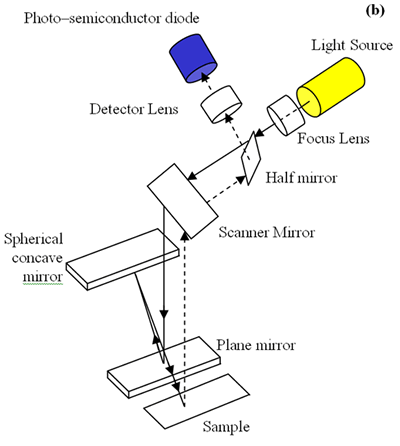 | Figure 1. (a) Basic concept of the profile measurement; (b) Schematic diagram of 3-D surface profiler. | The angle between the incident light and the reflected light is approximated to 2θ, which corresponds to the deviation Δ from the incident light beam. Under such condition, Δ is approximated to 2θl. When the deviation Δ is measured by scanning the object, the distribution function of the surface profile f(x) can be obtained by the measurement of Δ(x). From the equation (1), f(x) can be derived by: (2) where α = 1/2l. The coefficient α can be determined by a calibration measurement with a known object. Sequential scanning with the two–dimensional directions can provide the 3-D surface profile. The contrivance for surface profiler is illustrated in Figure 1(b). In this surface profiler, a scanner mirror is used instead of a scanning detector. This surface profiler was devised in a way that a spherical concave mirror was combined for the purpose of synchronizing the curvature with the optical intensity at the measured point. Experimental Corning–1737F (Corning Co.) glass was used as a substrate. As a photoalignment layer, we used a PI–VA film [1wt%, Nissan Chemical] that originally exhibited homeotropic alignment. This material was spin-coated on the glass substrates and then baked at 200°C for 1 hour. The chemical formulation of this polyimide is not available because of commercial confidentiality. The PI–VA film was exposed with unpolarized UV light (λ = 320 nm) using UV light guide (Suncure 202, Asahi Glass Company) at normal incidence (Figure 2). The periodical striped patterns were produced by means of a photomask (20 μm for fabrication of a cell and 100 μm for surface profiler measurement). The intensities of the UV light after passing through the 20 μm and 100 μm photomasks are 60 mW/cm2 and 70 mW/cm2, respectively. The exposure time was set to be 30 min. To verify the flatness of the PI–VA film, atomic force microscope (AFM) (Shimadzu, SPM–9500J2) was used. Exposure patterns for alignment will be alternating exposed (random planar) and unexposed (homeotropic) stripes. To examine these alignment patterns, LC cells with a cell gap of 5 μm were made using one exposed and another unexposed substrates. The cells were filled with 4′-n-pentyl-4-cyanobiphenyl (5CB, supplied by Merck) by capillary action in the isotropic phase and cooled to room temperature. The LC alignment of the cell was checked by optical polarizing microscope (OPM). For evaporation, 4′-n-pentyl-4-cyanobiphenyl (5CB, supplied by Merck) [TNI =35.5°C] was heated on a hot plate at 90°C and was adsorbed on the substrate surface positioned 50 mm from the LC source at room temperature in air. 5CB adsorption was controlled by the duration of evaporation. The alignment process of adsorbed LC multilayers on the surface of photoinduced PI–VA alignment film can be imagined as a microscopic image by means of a novel 3-D surface profiler instrument (Scanning Laser Imaging Scope, Core System Co. Ltd.). The remarkable features of this instrument are its wide range of scanning area (~ 40 mm) and high vertical resolution (~ 0.01 nm). 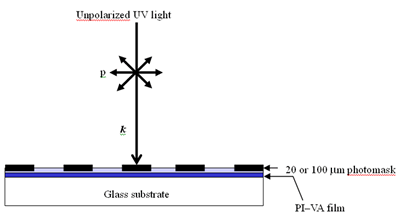 | Figure 2. Method of exposure to unpolarized UV light. Here p stands for the randomly oriented polarization directions of the incident unpolarized UV light and k denotes the propagation vector. | Results and Discussion LC Alignment Behavior of a Photoinduced PI–VA Film Figure 3 shows polarized microphotographs of a photoaligned LC cell between crossed polarizers. The alternating exposed and unexposed striped patterns can be clearly seen. The exposed region produces a random planar alignment with nematic schlieren texture. In the middle of each exposed region, there is a uniform planar line because this black line became bright, yielding hybrid orientation at 45° between crossed polarizers (Figure 4). In the unexposed region, the LC alignment is homeotropic since extinction is observed between the crossed polarizers. These alignment patterns can be imagined with the aid of Figure 5. The results are in good agreement with the previous elucidation of photodegradation effect. 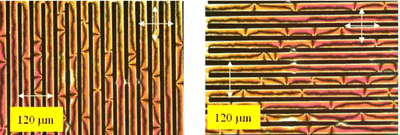 | Figure 3. Polarized microphotographs of a photoaligned LC cell between crossed polarizers. | 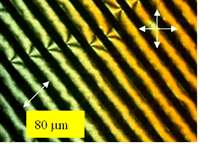 | Figure 4. Polarized microphotograph of a photoaligned LC cell at 45° between crossed polarizers. | 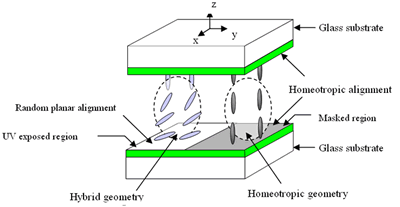 | Figure 5. The photoaligned LC cell having alternating exposed and unexposed striped patterns. | Surface Alignment of LC Molecules Evaporated on the Unexposed PI–VA Film Figure 6 shows a polarized microphotograph of the evaporated surface of the unexposed PI–VA film after 3 hours evaporation. Under crossed polarizers, very tiny homeotropic droplets (of the order of μm) were found due to the dewetting phenomena. This occurs when the surface energy of the PI–VA film is less than the sum of the interfacial energy between PI–VA and LC films and the surface energy of LC film. Figure 7 shows 3–D surface height pattern mapping of the LC evaporated surface of unexposed PI–VA film after 3 hours evaporation. From this pattern mapping, it was also found that the height of the surface topological roughness seems to be less than 0.1 nm. These results suggest that LC molecules were not adsorbed on the unexposed PI–VA film surface for sufficient time span of 3 hours evaporation and the surface density of LC molecules is quite low i.e. the growth of the first adsorbed LC monolayer cannot take place during this 3 hours time span, resulting in the formation of very small droplets probably due to dewetting phenomena [30-32]. 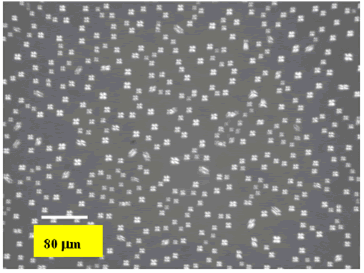 | Figure 6. Polarized microphotograph of the evaporated surface of unexposed PI–VA film for 3 hours evaporation under crossed polarizers. | 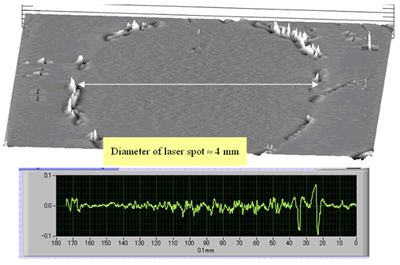 | Figure 7. 3–D surface height pattern mapping of the evaporated surface of unexposed PI–VA film for 3 hours evaporation. | Surface Alignment of LC Multilayers Evaporated on the Photoinduced PI–VA Film To verify the flatness of the PI–VA film, dynamic mode AFM images of photoinduced PI–VA film were taken. 20 μm photo–mask was used instead of 100 μm photo–mask because the scanning range of the AFM is 30 × 30 μm2. Figure 8 shows that the surface of the photoinduced PI–VA alignment film did not reveal periodical surface relief gratings but had a surface topology with a few angstroms surface roughness. 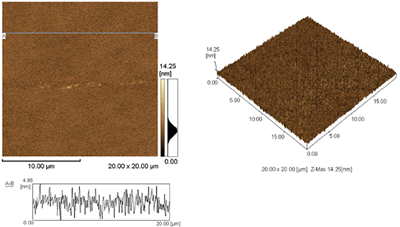 | Figure 8. 20 × 20 μm2 dynamic mode AFM images of the surface of photoinduced PI–VA alignment film. (a) 2–D display of the AFM data; (b) 3–D display of the AFM data. | Figure 9 and 10 show 3–D surface height pattern mappings of the evaporated surface of unpolarized UV–exposed (with 100 μm photomask) PI–VA film for 2 hours and 3 hours evaporation, respectively. At both 2 hours and 3 hours 5CB evaporation, a periodic profile can be clearly seen, where the period corresponds to the photomask pattern (100 μm line and space). Based on the result of Figure 7, it was recognized that the height of UV exposed region is greater than that of the masked region. This result implies that the surface molecular alignment at the UV exposed region is voluminous compared with the masked region. On the other hand, it is known from the measurement of LC cell alignment (Figure 3) that the surface LC molecules are randomly oriented in the UV exposed region due to the selective photodecomposition of the alkyl branches when the PI–VA film is exposed to unpolarized UV light. In order to validate these results, Figure 11(a) and (b) show polarized microphotographs of the evaporated surface of unpolarized UV–exposed PI–VA film for 2 hours and 3 hours evaporation, respectively. Figure 12 is the magnification of Figure 11(b). Periodic images can also be clearly seen in Figures 11 and 12. In the UV exposed region, a random planar alignment was found whereas homeotropic droplets appeared in the masked region. 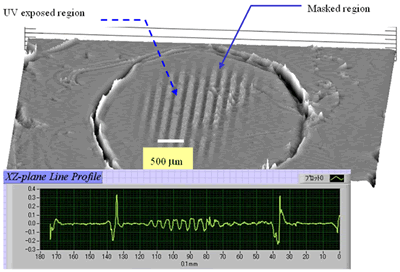 | Figure 9. 3–D surface alignment pattern mapping of the evaporated surface of unpolarized UV–exposed PI–VA alignment film taken by the surface profiler for two hours evaporation. Vertical height information: ≈ 0.16 nm (max.) for UV exposed region and a few angstrom surface roughness for the masked region. | 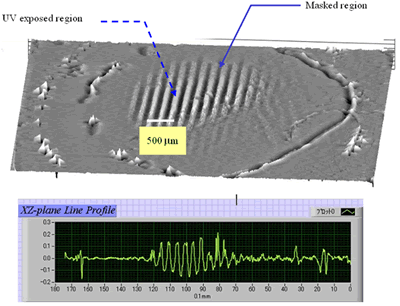 | Figure 10. 3–D surface alignment pattern mapping of the evaporated surface of unpolarized UV–exposed PI–VA alignment film taken by the surface profiler for three hours evaporation. Vertical height information: ≈ 0.28 nm (max.) for UV exposed region and a few angstrom surface roughness for the masked region. | 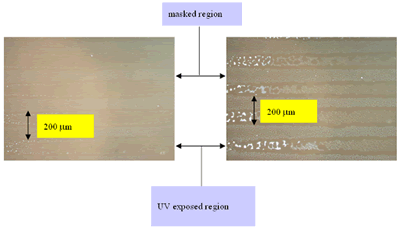 | Figure 11. Polarized microphotographs of the evaporated surface of unpolarized UV–exposed PI–VA alignment film for (a) 2 hours (b) 3 hours evaporation, under crossed polarizers. | 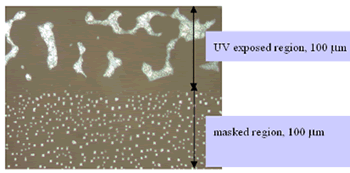 | Figure 12. Magnification of Figure 11(b) under crossed polarizers. | Conclusions The following experimental findings were made by the novel surface profiler instrument and the optical polarizing microscope. • A random planar alignment was realized by irradiation of normal incident unpolarized UV light on a photoalignment PI–VA film that originally exhibited homeotropic alignment. • The surface alignment of LC multilayers evaporated on the photoaligned PI–VA film exhibited a periodic profile. • The height of LC multilayers in the UV exposed region was greater than that in the masked region. This height difference revealed the different alignment mechanisms for the exhibited periodic profile. • By means of the optical polarizing microscopy, the random planar alignment in the UV exposed region and homeotropic droplets in the masked region were found and observed. • The photoinduced PI–VA film surface can align an adsorbed LC monolayer and then the alignment can be extended to the bulk via the epitaxylike LC–LC interaction i.e., a short–range molecular interaction. • For the unexposed PI–VA film surface, noticeable anisotropy in the monolayer alignment was not observed, indicating that the long–range elastic interaction may be responsible for the bulk alignment. • The appearance of very small droplets in the masked region may be presumably related to dewetting phenomena. The surface alignment of LC multilayers evaporated on commercially used polyimide film has been demonstrated by means of the novel surface profiler, opening a new area of research in surface alignment of LCs. Furthermore, it is shown that the open–air LC deposition on the patterned alignment is a potential technique to realize a functional surface. Acknowledgements This work was partially supported by a Grant–in–Aid for Encouragement of Young Scientists (B) (No. 15760020) from Japan Society for the Promotion of Science and 21st century COE program “Creation of Hybridized Materials with Super–Functions and Formation of International Research and Education Centre” from Ministry of Education, Culture, Sports, Science and Technology, Japan. References 1. A. A. Sonin, “The Surface Physics of Liquid Crystals”, Gordon and Breach, Amsterdam (1995) pp.x-xi. 2. B. Jérôme, “Surface effects and anchoring in liquid crystals”, Rep. Prog. Phys., 54 (1991) 391–451. 3. W. M. Gibbons, P. J. Shannon, S. –T. Sun and B. J. Swetlin, “Surface–mediated alignment of nematic liquid crystals with polarized laser light”, Nature, 351 (1991) 49–50. 4. M. O’Neill and S. M. Kelly, “Photoinduced surface alignment for liquid crystal displays”, J. Phys. D: Appl. Phys., 33 (2000) R67–R84. 5. K. Okano, N. Matsuura and S. Kobayashi, “Alignment of a Liquid Crystal on an Anisotropic Substrate”, Jpn. J. Appl. Phys., 21 (1982) L109–L110. 6. W. Chen, M. B. Feller and Y. R. Shen, “Investigation of Anisotropic Molecular Orientational Distributions of Liquid–Crystal Monolayers by Optical Second–Harmonic Generation”, Phys. Rev. Lett., 63 (1989) 2665–2668. 7. M. B. Feller, W. Chen and Y. R. Shen, “Investigation of surface–induced alignment of liquid–crystal molecules by optical second–harmonic generation”, Phys. Rev., A 43 (1991) 6778–6792. 8. D. W. Berreman, “Solid Surface Shape and the Alignment of an Adjacent Nematic Liquid Crystal”, Phys. Rev. Lett., 28 (1972) 1683–1686. 9. D. W. Berreman, “Alignment of Liquid Crystal by Grooved Surfaces”, Mol. Cryst. Liq. Cryst., 23 (1973) 215–231. 10. S. Faetti, “Azimuthal anchoring energy of a nematic liquid crystal at a grooved interface”, Phys. Rev., A 36 (1987) 408–410. 11. J. M. Geary, J. W. Goodby, A. R. Kmetz and J. S. Patel, “The mechanism of polymer alignment of liquid–crystal materials”, J. Appl. Phys., 62 (1987) 4100–4108. 12. D. Johannsmann, H. Zhou, P. Sonderkaer, H. Wierenga, B. O. Myrvold and Y. R. Shen, “Correlation between surface and bulk orientations of liquid crystals on rubbed polymer surfaces: Odd–even effects of polymer spacer units”, Phys. Rev., E 48 (1993) 1889–1896. 13. H. Kikuchi, J. A. Logan and D. Y. Yoon, “Study of local stress, morphology, and liquid–crystal alignment on buffed polyimide surfaces”, J. Appl. Phys., 79 (1996) 6811– 6817. 14. J. Cheng and G. D. Boyd, “The liquid–crystal alignment properties of photolithographic gratings”, Appl. Phys. Lett., 35 (1979) 444–446. 15. R. Barberi, I. Dozov, M. Giocondo, M. Iovane, Ph. Martinot–Lagarde, D. Stoenescu, S. Tonchev and L. V. Tsonev, “Azimuthal anchoring of nematic on undulated substrate: Elasticity versus memory”, Eur. Phys., J. B 6 (1998) 83–91. 16. J. Cognard, “Alignment of Nematic Liquid Crystals and Their Mixtures”, Mol. Cryst. Liq. Cryst., (Suppl. Ser.) A5 (1982) 1–74. 17. B. Jérôme, “Surface Alignment”, in Handbook of Liquid Crystals, 1 (Ed. D. Demus, J. Goodby, G. W. Gray, H. –W. Spiess and V. Vill), Wiley, New York (1998) pp. 535 –548. 18. M. Barmentlo, R. W. J. Hollering and N. A. J. M. van Aerle, “Correlations between surface and bulk liquid–crystal alignment observed with optical second–harmonic generation”, Phys. Rev., A 46 (1992) R4490–R4493. 19. Y. R. Shen, “Surface properties probed by second–harmonic and sum–frequency generation”, Nature, 337 (1989) 519–525. 20. Y. R. Shen, “Optical second-harmonic generation at interfaces”, Annu. Rev. Phys. Chem., 40 (1989) 327–350. 21. C. S. Mullin, P. Guyot–Sionnest and Y. R. Shen, “Properties of Liquid–Crystal Monolayers on silane surfaces”, Phys. Rev., A 39 (1989) 3745–3747. 22. G. T. Boyd, Y. R. Shen and T. W. Hänsch, “Continuous–wave second–harmonic generation as a surface microprobe”, Opt. Lett., 11 (1986) 97–99. 23. K. Pedersen and S. I. Bozhevolnyi, “Second–Harmonic Generation Scanning Microscopy on Domains in Al Surfaces”, Phys. Stat. Sol., (a) 175 (1999) 201–206. 24. R. Shinozaki, O. Sasaki and T. Suzuki, “Fast scanning method for one-dimensional surface profile measurement by detecting angular deflection of a laser beam”, Appl. Opt., 43 (2004) 4157–4163. 25. T. N. Oo, Y. Ohta, N. Tanaka, T. Iwata, M. Kimura and T. Akahane, “Study of surface alignment of liquid crystal multilayers evaporated on polyvinylcinnamate photoalignment film”, Adv. in Tech. of Mat. and Mat. Proc. J. (ATM), 7 [1] (in press). 26. M. Hasegawa and Y. Taira, “Nematic Homogeneous Photoalignment by Polyimide Exposure to Linearly Polarized UV”, J. Photopolym. Sci. Technol., 8 (1995) 241–248. 27. H. Yoshida and Y. Koike, “Inclined Homeotropic Alignment by Irradiation of Unpolarized UV light”, Jpn. J. Appl. Phys., 36 (1997) L428–L431. 28. H. F. Li, E. J. Kim, H. Furue, Y. Iimura and S. Kobayashi, “Control of Pretilt Angle and its Direction in Homeotropic Alignment of Liquid Crystals Using Photoalignment Technique”, Mol. Cryst. Liq. Cryst., 326 (1999) 249–257. 29. Y. Iimura and S. Kobayashi, “Prospects of the Photo–Alignment Technique for LCD fabrication”, SID 97 DIGEST, (1997) 311–314. 30. M. Woolley, R. H. Tredgold and P. Hodge, “Ultrathin Films of Smectic Liquid Crystals on Solid Substrates”, Langmuir, 11 (1995) 683–686. 31. M. Bardosova and R. H. Tredgold, “Ultra–Thin Films of Smectic Liquid Crystals on Solid Substrates”, Mol. Cryst. Liq. Cryst., 355 (2001) 289–303. 32. I. Drevenšek Olenik, K. Kočevar, I. Muševič and Th. Rasing, “Structure and polarity of 8CB films evaporated onto solid substrates”, Eur. Phys. J., E 11 (2003) 169–175. Contact Details |