Introduction Sialons are ceramics phases occurring in the SiO2 – Si3N4 – AlN – Al2O3 system. Due to their chemical inertness, good thermal shock resistance, and excellent mechanical properties retained up to high temperatures, sialon systems have found considerable applications in engineering [1]. They can have a wide range of compositions, the more commonly encountered forms of sialon being β-sialon, O-sialon, X-sialon, and α-sialon. β-sialon is Si6–zAlzOzN8–z, where z can have values from 0 (pure Si3N4) to 4.2. These sialons are isostructural with β-Si3N4. O-sialon is Si2 – xAlxO1+xN2 – x, where x can have values from 0 to about 0.4. X-sialon, nominally Si12Al18O39. These sialons are isostructural with mullite, and can be regarded as solid solutions of mullite with Si3N4. α-sialon is isostructural with α-Si3N4 and can be formed in the presence of stabilizing metal ions as Mg, Y or Ca [1]. Sialons are almost never found as natural minerals and sialon powders must be synthesized. Sialons can be made by reaction sintering or hot pressing a starting powder mixture of oxide, nitride and oxynitride powders [1, 2]. They can also be made using of a carbothermal reduction process (CTR) [3, 4]. On the other hand, reactive dc magnetron sputtering is a technique that allows the development of various types of coatings. These coatings can be pure metallic and non-metallic, metallic oxides, metallic nitrides and metallic carbides; with very varied structures and properties [5]. There are few reports in the literature on the manufacture of coatings from the quaternary Si-Al-O-N system [6]. In this work we report studies on coatings from this system, using different techniques to characterize their structure and chemical composition. Experimental Coatings from the Si – Al – O – N system were prepared using DC magnetron reactive co-sputtering technique (Sputtering INTERCOVAMEX system) on high speed steel (HSS) substrates. A cylindrical aluminum tablet of 5.0 cm diameter was used as the target, which was covered partially with pieces of c-Si, so that Si:Al fractions 50:50 and 60:40 in area were defined. Argon was used as the like inert gas (99.999% purity), and oxygen and nitrogen (99.999% and 99.5% purity, respectively) as the reactive gases. Optical emission spectroscopy (OES) was performed during the growth processes of the coatings, in order to detect the involved species in the plasma (optical fiber spectrometer Ocean Optics 2000). The resultant coatings were analyzed by scanning electron microscopy (SEM) (PHILIPS EDAX XL30 ESEM). Additionally, analyses of chemical composition by energy dispersive spectroscopy (EDS) were performed for each sample. Structure was studied by X-ray diffraction (XRD, Rigaku D/Max-2100). Micro-Raman dispersion spectra were obtained using a DILOR Spex system, working with the He-Ne excitation line at 632.8 nm. The parameters used in the growth process of the coatings obtained are listed in Table 1. Table 1. Growth parameters of reactive DC magnetron sputtering system for the Si-Al-O-N system. Coating | SO3N10B0 | 60 | 25 | 3 | 10 | 1.0 x 10-1 | 35 | 0 | SO3N12B0 | 60 | 25 | 3 | 12 | 1.0 x 10-1 | 33 | 0 | SO0N20B0 | 60 | 20 | 0 | 20 | 8.8 x 10-2 | 30 | 0 | SO0N20B-300 | 60 | 15 | 0 | 20 | 7.6 x 10-2 | 35 | -300 | SO0N18B-300 | 60 | 18 | 0 | 18 | 8.0 x 10-2 | 30 | -300 | SO0N20B-250 | 50 | 15 | 0 | 20 | 1.2 x 10-1 | 30 | -250 | Results and Discussion The objective of this work was to develop a production method for coatings from the Si-Al-O-N system by means of the reactive DC magnetron sputtering technique. Coatings were obtained by varying growth parameters (Table 1), allowing us to evaluate the effect on structure and composition of the samples. The EDS chemical compositions of each of the obtained coatings are listed in Table 2. Table 2. Chemical composition of the coatings samples obtained by EDS measurements. Coating | SO3N10B0 | 25.51 | 13.03 | 61.45 | 0 | SO3N12B0 | 28.51 | 10.41 | 61.08 | 0 | SO0N20B0 | 40.69 | 4.48 | 51.78 | 3.05 | SO0N20B-300 | 35.70 | 8.30 | 49.08 | 6.92 | SO0N18B-300 | 42.29 | 4.85 | 47.66 | 5.20 | SO0N20B-250 | 40.30 | 3.89 | 49.36 | 6.45 | It is seem that the bias voltage in the substrate aids nitrogen incorporation. Without bias voltage and with a nitrogen flow of 20 sccm, a coating contains 3.05 at.% of nitrogen; on the other hand, 6.45 and 6.92 at.% of nitrogen content are obtained, respectively, when bias voltages of -250V and -300V are applied. Nevertheless, the application of high negative bias voltages to the substrate produces strong erosion of the coating (Figure 1). 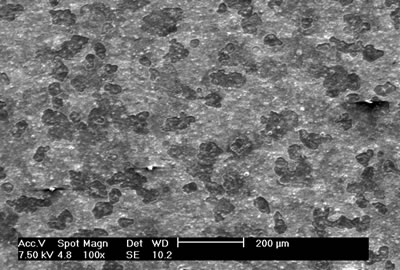 Figure 1. Scanning electron microscopy image of SO0N20B-250 coating obtained using a -250 V bias voltage on the substrate. When bias is not applied, no erosion of the surface is observed in Figure 2. 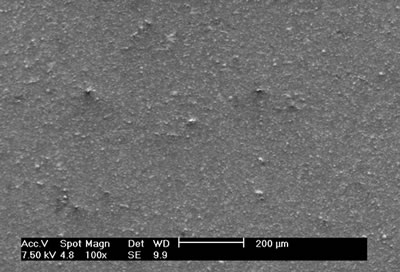 Figure 2. Scanning electron microscopy image of SO0N20B0 coating obtained without bias voltage on the substrate. The optical emission spectrum of sample SO0N20B-250 (Figure 3) shows the following species: Ar(I), which are the most remarkable lines; N2, N2+, N(I), Al(I) and Si(II), at 375 nm, 391nm, 868 nm, 394 nm and 637 nm, respectively [7, 8]. 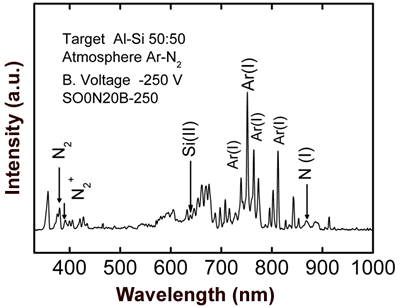 Figure 3. Optical emission spectrum obtained during evaporation of SO0N20B-250 coating. Figure 4 shows OES spectra of the co-sputtering process with an aluminum-silicon target. All curves show characteristic peaks of Argon (20 sccm); the bottom curve is due to a process with a pure argon atmosphere, where we can clearly see the signal of aluminum (396 nm). The other curves were obtained introducing different fluxes of nitrogen (5, 10, and 15 sccm) in the chamber vacuum in addition to argon. We can observe the nitrogen peaks (N2, N2+ and N (I)) increasing and a reduction in the argon peaks when the nitrogen flux increases. We observe also that there is no effective aluminum erosion (absence of the Al peak) when nitrogen flux is introduced into the vacuum chamber, which can be due to nitridation of the aluminum-silicon target [9]. In order to avoid complete nitridation of the target and to obtain Al evaporation, in accord with S. Berg [9], it is necessary to monitor the emission of Al when the nitrogen flux gradually increases. 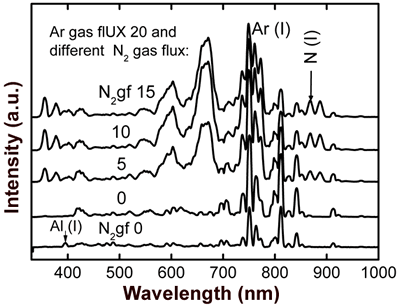 Figure 4. Optical emission spectra obtained through aluminum-silicon target sputtering in metallic mode (using pure argon) for the lower curve, and reactive mode (using argon-nitrogen) for the upper curves. A typical X-ray diffraction pattern of sample SO0N20B-250 is shown in Figure 5. This pattern shows polycrystalline Si peaks [JCPDS powder diffraction file 27-1402] and a wide peak at about 22º due to an amorphous structure. This result indicates that this sample is a composite material, a mixture of amorphous Si-Al oxynitride and nanocrystalline Si, which we know has low Al and nitrogen concentrations. 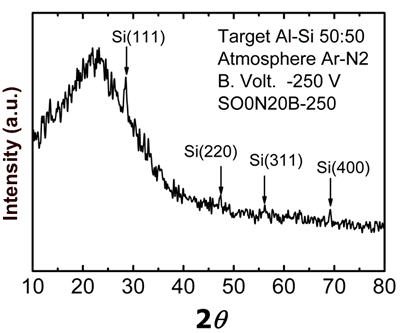 Figure 5. X-ray diffraction pattern of SO0N20B-250 coating. Considering that the sample is a composite material, we performed measurements in three representative regions selected by the optical microscope of the micro-Raman system (Figure 6). Region 1 (R1) corresponds to micro-hills features, while regions 2 (R2) and 3 (R3) correspond to micro-holes and the dominant region of the surface, respectively. 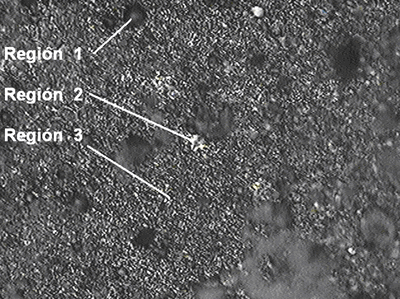 Figure 6. Optical micrograph of SO0N20B-250 coating, where the selected regions for micro-Raman scattering measurements are shown. Micro-Raman scattering spectra of the SO0N20B-250 coating for each one of the former regions are shown in Figure 7. The three spectra are similar curves, but R2 and R3 curves show more intense peaks. 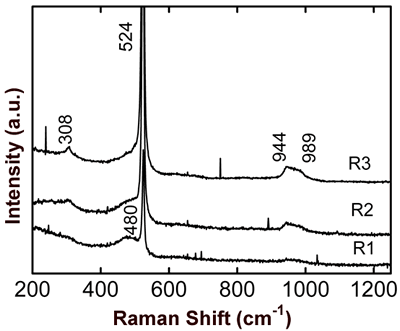 Figure 7. Micro-Raman scattering spectra for three representative regions of SO0N20B-250 coating. The main peak in the spectra is at about 521 cm-1, and there is a weak peak at about 308 cm-1 corresponding to Si crystals [10]. The peak at 521 cm-1 has a full width at half maximum (FWHM) of about 6.2 cm-1. Data fitting of this peak to a model of Si spherical grains indicates a grain size about 200 nm. This peak is shifted for 3.3 cm-1 with respect to an unstressed Si crystal. This is a gross extrapolation of results reported in [11], due to a compressive stress of about 1.4 GPa. In view of the content of nitrogen in the coatings of about 3-7 at.%, it is possible that some Si grains are partially nitrided to form SiNx [12]. A broad and weak band observed at about 480 cm-1 is due to amorphous silicon [10]. It is known that vitreous silica has a signal at about 1000 – 1150 cm-1 [13], but in these spectra it is not observed. From 930 to 1000 cm-1 there is signal associated with Al-O-Si antisymmetric vibrations [13]. Conclusions In this work we controlled three main evaporation parameters to produce Si-Al-O-N coatings: oxygen flux, nitrogen flux and substrate bias potential. Oxygen incorporation into the coatings can be produced even though on oxygen flux is absent. The introduction of nitrogen gas into the vacuum chamber allows the formation of nitride coatings, but also of nitrides at the surface of the aluminum target. During co-sputtering of the Al-Si target with an atmosphere of argon-nitrogen, Al evaporation is partially inhibited by the nitrogen presence due to a thin film of AlN appearing on the surface of the target. In spite of high negative bias voltage producing severe erosion on the coatings, it improves nitrogen adsorption into the coatings. Micro-Raman scattering and X-ray diffraction measurements allowed us to show that the coatings are composite materials of nanocrystalline Si-SiNx embedded in an amorphous Al-Si-O matrix. Acknowledgements The authors acknowledge the technical support of Pedro García Jiménez, José Eleazar Urbina Álvarez, Martín Adelaido Hernández Landaverde, Francisco Rodríguez Melgarejo, Agustín Galindo Sifuentes, Rivelino Flores Farías, and Ma. del Carmen Delgado Cruz. Also, we acknowledge the help given by S. Jimenez Sandoval to interpret some results. R. A. Vargas acknowledges the scholarship assigned by CONACyT during his M. C. program. This work was supported by CONACyT-Mexico. References 1. K.H. Jack, “Sialons and Related Nitrogen Ceramics”, J. Mater. Sci., 11 (1976) 1135-1158. 2. W.E. Lee and W.M. Rainforth, “Ceramic Microstructures”, Chapman and Hall, London (1994), pp. 388-411. 3. X.-J. Liu, X.W. Sun, J.J. Zhang, X.P., Pu, Q.M. Ge, and L.P. Huang, Mater. Res. Bull., 38 (2003) 1939-1948. 4. M. Panneerselvam and K.J. Rao, Mater. Res. Bull., 38 (2003) 663 – 674. 5. K. Wasa, S. Hayakawa, “Handbook of Sputter Deposition Technology”, Noyes Publications, New York (1992) pp. 24,118, 125-133. 6. M Jacobs and F. Bodart, “Depth Profiles in Nitrogen Implanted SixAly Coatings Post-Implanted with Oxygen”, Surface and Coating Technology, 103-104 (1998) 113-117. 7. D.R. Lide, “CRC Handbook of Chemistry and Physics”, Editor-In-Chief, 80th Edition, Boca Raton, Florida (1999-2000). 8. S. Meter, F. Richter, R. Tabersky, and U. König. “Optical Emission Spectroscopy of a PCVD Process Used for the Deposition of Ti Non Cemented Carbides”. Thin Solid Films, 377-378 (2000) 430-435. 9. S. Berg, T. Larsson, and H-O. Blom, “The Use of Nitrogen Flow as a Deposition Rate Control in Reactive Sputtering”, J. Vac. Sci. Technol., A 4 (1986) 594-597. 10. Y. Kanzawa, S Hayashi and K. Yamamoto, “Raman Spectroscopy of Si-rich SiO2 Films: Possibility of Si Cluster Formation”. Condens. Matter, 8 (1996) 4823 – 4835. 11. I. De Wolf, “Stress Measurements in Si Microelectronics Devices Using Raman Spectroscopy”, J. Raman Spectroscopy, 30 (1999) 877-883. 12. V. A. Volodin, M. D. Efremov, V.A. Gritsenko, and S. A. Kochibei, “Raman Study of Silicon Nanocrystals Formed in SiNx Films by Excimer Laser or Termal Annealing”, Appl. Phys. Lett., 73 (1998) 1212-1214. 13. R. Le Parc, B. Champignon, J. Dianoux, P. Jarry, and V. Martinez. “Anorthite and CaAl2Si2O8 Glass: low Raman Spectroscopy and Neutron Scattering”, Journal of Non-Crystal Solids, 323 (2003) 155-161. Contact Details |