Silicate residues are a significant group of materials when considering the recycling and reuse of industrial waste. They include coal power station ash, bottom-ash and fly ash from waste incinerators, slag from steel production, and glass cullet and combustion dusts. A conventional method for the recycling and reuse of these waste materials is vitrification - melting the residues at high temperatures (1,300-1,500°C) to produce homogeneous glass. However, vitrification is an energy-intensive, relatively costly process. Therefore, its use can only be fully justified and commercially viable if high quality products can be made that compete with current materials. Our latest work at Imperial College is addressing this requirement, making useful products that have numerous potential applications. Glass-Ceramics The properties of standard vitrified products are insufficient for architectural applications and structural building components, insulation or other specialised applications. Yet there is an effective way to improve these properties without major alterations to the process itself - the introduction of a controlled crystallisation process through a subsequent heat treatment, i.e. by forming a glass-ceramic. Production of Glass-Ceramics Glass-ceramic articles may be produced by three routes: • The heat treatment of solid glass (the traditional route) • The controlled cooling of a molten glass, known as the petrurgic method • The sintering and crystallisation of glass powders. In the latter case, the powders are densified at relatively low temperatures by exploiting a viscous flow sintering mechanism. After densification, the material is subjected to a crystallisation heat-treatment to obtain the required glass-ceramic microstructure. Alternatively, both densification and crystallisation may take place during a single sintering step. Along with the economic advantage of using relatively low processing temperatures, the powder technology route is suitable for the production of a range of advanced materials, including glass-ceramics with specified porosities and glass-ceramic matrix composites. Using the petrurgic method, the slow cooling from the molten state causes nucleation and growth of certain crystalline phases. Therefore, the final microstructure, and hence the properties, depends mainly on the composition and the cooling rate. Glass-Ceramics Based on Coal Ash The very high iron oxide content of coal ash, table 1, indicates the potential for developing magnetic phases using appropriate processing - this was the aim of our work. We calcined the as-received ash at 800°C for two hours to remove any volatile compounds, including sulfur and carbon. The powder and petrurgic methods were explored, and gave us products with different phases and microstructures. For the sintering experiments, we mixed calcined ash powder with various amounts (10-50wt%) of borosilicate (Pyrex) glass. The powder mixtures were uniaxial cold pressed to a cylindrical shape and sintered in air at temperatures in the range of 1,000-1,500°C for periods of up to 15 hours. Using the petrurgic method, coal ash was mixed with soda-lime glass powder. The mixture was melted at 1,500°C and cooled to room temperature at rates of between 1-10°C per minute. Table 1. Chemical composition (wt%) of the investigated coal and incinerator ash. | Fe2O3 | 43.5 | Na2O | 3.5 | SiO2 | 31.0 | MgO | 2.4 | Al2O3 | 11.4 | Al2O3 | 17.5 | CaO | 4.0 | SiO2 | 38.0 | TiO2 | 2.3 | P2O5 | 1.6 | ZnO | 1.4 | SO3 | 0.2 | MgO | 1.3 | Cl2 | - | Cr2O3 | 0.9 | K2O | 1.8 | Alkalis | 2.2 | CaO | 21.1 | Traces | Ni, Cu, etc | TiO2 | 1.7 | | | Cr2O3 | - | | | MnO | 0.4 | | | Fe2O3 | 8.0 | | | NiO | - | | | ZnO | 3.5 | Structure and Properties The microstructure of a glass-ceramic containing equal proportions of borosilicate glass and coal ash, obtained by sintering for five hours at 1,500°C revealed that the addition of borosilicate glass proved successful in promoting densification by viscous flow, resulting in a highly dense product. The sintering process was optimised so that no ‘barrelling’, swelling or other shape distortion effects occurred, and the samples retained their original cylindrical form. This is particularly important when fabricating components of complex shapes and good dimensional tolerances for technical applications. The sintered materials exhibit a well-developed and reproducible glass-ceramic microstructure, comprising a silicate matrix with dispersed crystalline phases. The matrix was B2O3-Fe2O3-CaO-SiO2-Al2O3 glass with one of the crystalline phases enriched in metals, especially iron and titanium. This we identified as a ferrite-type phase. The most interesting feature of these samples is their magnetic behaviour. The magnetisation curves are characteristic of soft ferromagnetic materials, with magnetisation saturating at low fields. In our samples, the saturation magnetisation was nearly 6 emu/g, the remnant magnetisation was 3 emu/g and the coercitivity was ~540 Oe. Coal Ash Based Glass-Ceramics produced by the Petrurgic Process Figure 1 shows the typical microstructure of a glass-ceramic obtained by the petrurgic method, which was produced from a melt containing 60wt% soda-lime glass and 40wt% coal ash at a cooling rate of 10°C per minute. The sample has a well-developed dendritic structure, ascribed to magnetite, which confers soft magnetic properties to the material. 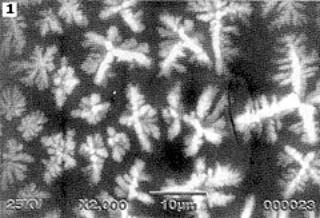 | Figure 1. SEM micrograph of 60wt% soda lime glass, 40wt% coal ash, fabricated using the petrurgic method (cooling rate 10°C/min) | Results demonstrate the potential of both the sintering and petrurgic routes to fabricate glass-ceramic materials with soft magnetic properties from iron-containing silicate residues. Works in Progress Our latest experiments are designed to assess quantitatively the dependence of magnetic properties from the magnetic phase content and other microstructural features in these glass-ceramics. The aim is to determine if better soft magnetic or unique magnetic behaviour can be achieved by adjusting the processing conditions, which would then enable a variety of technical applications for the products. Incinerator Fly Ash Based Glass-Ceramics In addition to the oxides, table 1, we detected traces of tin, antimony, cadmium, barium, arsenic, strontium, zirconium, lead and molybdenum in the incinerator filter ash. The traditional route involved melting the as-received ash at 1,400°C for two hours, quenching in air at room temperature, and subsequent heat-treatment at pre-determined temperatures in the range of 850-950°C to induce nucleation and crystal growth. Structure and Properties The microstructure of the glass-ceramic produced in this way consisted mainly of crystals of the pyroxene group (e.g. diopside) embedded in a glassy matrix. The sintering route involved milling the vitrified incinerator ash to produce a fine powder with an average particle size of 35µm. We made cylindrical compacts by uniaxial cold-pressing and subjected them to a two step heat treatment schedule that ensured sintering was largely completed before crystallisation started. The densified material shows a high degree of crystallisation, characterised by a network of interlocked elongated (axial ratio 3-5) crystals between which smaller, equiaxed crystals are situated, embedded in a residual glassy matrix, figure 3. 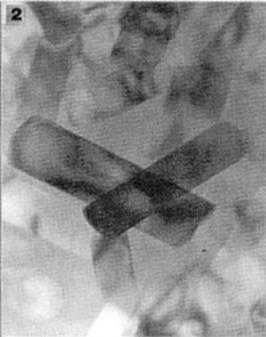 | Figure 2. TEM micrograph of the highly dense area of a highly crystalline glass-ceramic made by sintering vitrified fly ash, showing equiaxed nanocrystals | We measured the mechanical properties of both the melt-derived and sintered products. The data is compared with those for the as-quenched glass and for a hot-pressed borosilicate glass in table 2. Particularly important is the attainment of a relatively high hardness, fracture toughness and strength, as well as adequate machinability in our glass-ceramics. The simultaneous increase of hardness and toughness indicates improved wear resistance of the glass-ceramic in comparison with the as-quenched glass. The improvement of the thermal shock resistance, measured by the water-quenching method, is also worth noting. Table 2. Mechanical properties of sintered glass-ceramics. | Density (g/cm3) | 2.74±0.02 | 2.80±0.02 | 2.89±0.02 | 2.22 | Hardness (GPa) | 3.8±0.2 | 5.5±0.2 | 7.9±0.3 | 5.1±0.2 | Young’s Modulus (GPa) | 85±4 | 93±4 | 124±5 | 63 | Poisson’s ratio | 0.24 | 0.28 | 0.26 | 0.22 | Thermal exp. Co-eff (x10-6/°C) | 6.0 (20-700°C) | 5.9 (20-600°C) | 6.5 (20-700°C) | 3.3 (20-600°C) | Indentation Fracture Toughness MPa.m½) | 1.5±0.1 | 0.6±0.1 | 1.7±0.1 | 0.7±0.1 | Modulus of Rupture (MPa) | 88±9 | 90±10 | 240±20 | 60 | Thermal Shock Resistance (Water Quench Test, ΔTc, (K)) | ~300 | ~150 | ~280 | ~250 | Brittleness Index (µm-½) | 2.5 | 9.1 | 4.6 | 7.2 | Glass-Ceramic Composites Work to date has largely concentrated on composites with a matrix of the slag-based Silceram glass-ceramic (a glass-ceramic for floor and wall tiles and wear components). We have investigated both particulate- (SiC and TiC) and fibre-reinforcement (SiC). Properties measured include the fundamental mechanical properties but also more complex properties such as thermal shock resistance and erosion resistance. As mentioned previously, the thermal shock resistance of glass-ceramics is superior to the parent glasses, and the shock resistance is further improved by particulate reinforcement. For example, monolithic Silceram has a thermal shock critical temperature of 180°C, whereas a 20wt%SiC composite has a value of 270°C. Erosion resistance may also be improved by particulate reinforcement, e.g., for TiC reinforced Silceram - the larger the reinforcement particle size and the greater the volume fraction, the lower the erosion rate. Results indicate a way for transforming vitrified silicate residues into useful products with broad application potential. The glass-ceramics obtained are candidate materials for applications in floors of industrial buildings and in construction, and for outside and inside facing walls. We are currently addressing issues associated with the effect of environmental influences on the chemical durability and toxic potential of the materials, which may be compromised by the presence of heavy metals incorporated in the glass or crystalline phases. Public acceptance of the use and exploitation of glass-ceramic-based materials in such applications will strongly depend on a satisfactory consideration of these issues. |