If it can’t anchor, it can’t survive An organism that depends on filtering nutrients from flowing water must find a way to anchor itself against the flow. The Venus’ flower basket, a sponge, uses extraordinarily strong spicules — strands of nested glass columns — to do the trick.
Brown University Engineers have led a study that has shown that the hair-like appendages of the Venus’ flower basket sea sponge derive their strength from their remarkable internal structure. These tiny appendages, which are made of glass, enable the Venus’ flower basket to anchor itself to the sea floor. This natural internal structure could inspire engineered structures.
The hair-like appendages known as basalia spicules, are fine-tuned for strength. Each spicule has a diameter of only 50µm. It has a silica core, which is surrounded by approximately 10 to 50 concentric glass cylinders. A very thin layer of an organic material separates each of these cylinders. The thickness of the walls of each cylinder decreases in thickness from the center core to the outer edge of the basalia spicules.
This pattern of decreasing thickness caught the interest of Haneesh Kesari, an assistant professor of engineering at Brown University. Initially, he was not able to understand this structure.
It was not at all clear to me what this pattern was for, but it looked like a figure from a math book. It had such mathematical regularity to it that I thought it had to be for something useful and important to the animal.
Haneesh Kesari, an assistant professor of engineering at Brown University
In order to survive, these sea sponges have to anchor themselves to the sea floor. If they get cast about with the flow then they would not be able to survive. These sponges filter nutrients out of the water in order to sustain life. Hence Kesari thought that natural selection could have made the spicule anchors very strong. Further, the thickness pattern could be contributing to its strength.
“If it can’t anchor, it can’t survive,” Kesari said. “So we thought this internal structure must be contributing to these spicules being a better anchor.”
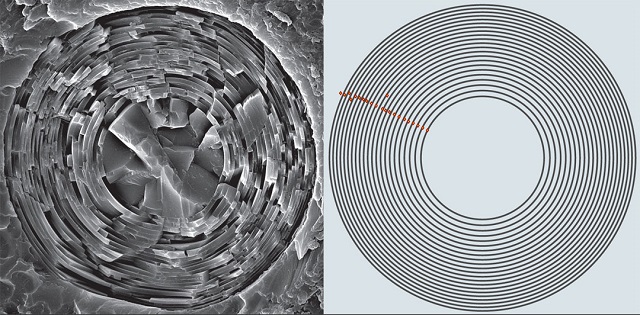
Image caption: Patterned for strength - A mathematical model, right, predicted that the thickness of nested glass cylinders should decrease from the core to the exterior to optimize strength. Studies of hundreds of spicules, left, showed that was exactly the case in nature.
Kesari and Michael Monn, a graduate student, built a mathematical model of the spicules’ structure to find out the reason. They assumed that glass cylinders would be able to slide against each other due to the organic layers between them.
We prepared a mechanical model of this system and asked the question: Of all possible ways the thicknesses of the layers can vary, how should they vary so that the spicule's anchoring ability is maximized?
Haneesh Kesari
The model proposed that the load capacity of the structure would be at maximum when the thickness of the layers decreased toward the outside. James Weaver and Joanna Aizenberg of Harvard’s Wyss Institute for Biologically Inspired Engineering conducted studies on this sponge species. Kesari and Monn then collaborated with Weaver and Aizenberg to compare the actual layer thicknesses in samples of the sponge’s spicules to the layer thicknesses that was predicted by the mechanics model.
The team found that the layer thicknesses observed in the samples and the model’s predictions matched very closely. “It appears that the arrangement and thicknesses of these layers does indeed contribute to the spicules’ strength, which helps make them better anchors,” Kesari said.
The researchers consider their study to be the first one to assess the mechanical advantage of this specific layer arrangement. Further, this arrangement could inspire useful engineered structures.
“In the engineered world, you see all kinds of instances where the external geometry of a structure is modified to enhance its specific strength — I-beams are one example,” Monn said. “But you don’t see a huge effort focused toward the internal mechanical design of these structures.”
The current study shows that the sponge spicules could act as a blueprint for making load-bearing beams stronger from their inner side to the outer side.
The researchers have published this study in the scientific journal, Proceedings of the National Academy of Sciences.
The National Science Foundation and the KIMM–Brown Nano and Micromechanics for Disaster Mitigation and Technological Reliability project have provided support for this project with grants.
References