Sponsored by HORIBAOct 24 2013
Raman micro-spectroscopy encompasses a broad range of activities and electrochemistry can profit from this technique to set up in situ analyses to track kinetic phenomena. A lithium polymer battery cycling mechanism is driven by the ionic transport in a polymer electrolyte (polyethylene Oxide (PEO) –Lithium salt) and the intercalation of Lithium in the cathode (V2O5). Raman micro spectroscopy provides information to monitor these properties in the batter.
Measure of the Salt Concentration in the Polymer Electrolyte
An electrolyte is a medium able to transport electric current using the ions it contains. The diffusion of an electrolyte is measured by the diffusion coefficient D (cm2s-1) and the migration is measured by the transport numbers t- and t+.
A number of techniques are available to measure those parameters in liquid electrolytes, however those techniques become uncertain in the case of polymer electrolytes. So Raman microspectroscopy appears as an alternative tool. In this instance, the electrolyte contains PEO heated to 80°C as the solvent in which the Lithium Li+ salt of tetra fluorosulfoneImide, TFSI- is solvated. At 80°C, PEO is completely amorphous and participates in the motion of Li+.
PEO contains a characteristic peak located at 1420 cm-1, which is assigned to the deformation, δ(CH2). The anion TFSI has a unique Raman signature with one characteristic peak which does not overlap with the Raman spectrum of PEO. This peak is the symmetric deformation vibration of the CF3 group (δs(CF3) at 742cm-1 as shown in Figure 1a. The relation between the ratio of the area corresponding to these peaks
A[δs(CF3)]/ A[δ (CH2)] is demonstrated to be linear in a wide range of concentrations as shown in Figure 1b.
Using this prime relation, ionic diffusion and ionic migration can be observed using Raman microspectroscopy as P(EO)n LiTFSI films.
![(a) Raman spectra of several P(EO)n LiTFSI concentrations. (b) Linear relation between relative area of TFSI peak - A[ds(CF3)]/ A[d (CH2)]- and concentration of TFSI in PEO.](https://d12oja0ew7x0i8.cloudfront.net/images/Article_Images/ImageForArticle_10128_45369335665381946226.jpg)
Figure 1. (a) Raman spectra of several P(EO)n LiTFSI concentrations. (b) Linear relation between relative area of TFSI peak - A[δs(CF3)]/ A[δ (CH2)]- and concentration of TFSI in PEO.
A concentration gradient is induced by the assembly of two polymers films (~100µm thick) with different LiTFSI salt concentrations at 80°C. Hence a diffusion of the salt from the higher to the lower concentration film can be observed by Raman microspectroscopy. A line of Raman analysis points is defined on the edge of the 2 films assembly to measure the concentration at several points of the cross-section as shown in Figure 2.
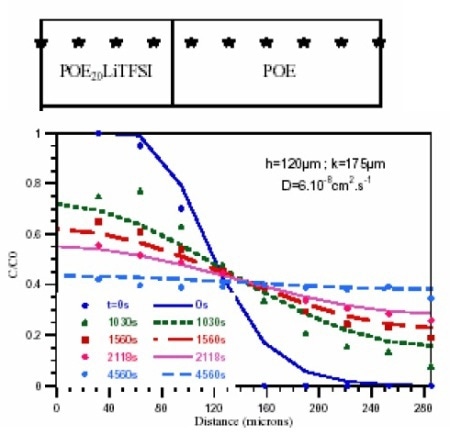
Figure 2. Evolution of the concentration profile of TFSI by self diffusion in the assembly consisting of a P(EO)20 LiTFSI and a PEO film at 80°C. Plain lines: theoretical function (with D=6.10-8cm2.s-1) Dots: experimental results.
Study of a Lithium/Polymer/Lithium Symmetric Cell
The Raman observation of the concentration profile while current densities are passing through a Lithium /polymer/lithium symmetric cell helps in determining the salt diffusion coefficient and ionic transport numbers.
Figure 3 displays the experiment performed on a Li/PEOLiTFSI/Li cell. Before current is applied certain lines are recorded to check the homogeneity of the concentration throughout the electrolyte. After that successive opposite constant currents are applied and the concentration gradient established in the electrolyte at the steady state can be measured.
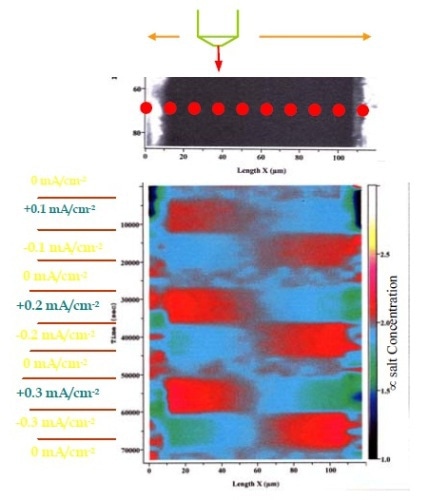
Figure 3. (a) Photograph of the PEO LiTFSI electrolyte between the lithium electrodes displaying the investigated line of points. (b) Image of the salt concentration -depending on position (x) and time (y)-
Study of the Intercalation and Release of Lithium in the Cathode Material LixV2O5
A large number of cathode materials have been considered. In this study, a cathode is analyzed that is mainly composed of V2O5 powder and a small quantity of carbon. Raman microspectrometry helps monitor the blend homogeneity.
The first intercalation of lithium in the initial cathode material α-V2O5 induces phase transitions to the ε, δ, γ and ω phases. The Raman spectrum of α- V2O5 has been thoroughly studied.
The Raman spectrum of each phase is recorded during the first discharge of a Li/electrolyte/V2O5 battery. The characteristic spectra of phases α, ε, ε', δ, γ are plotted in Figure 4. Each phase is characterized by a shift of the V-OA stretching mode located respectively at 995cm-1, 982cm-1, 972 cm-1 and 1006cm-1. The γ phase is unique by exhibiting two peaks at 865 and 915cm-1. No characteristic spectrum of the ω phase has been obtained as it seems that this phase does not provide any significant Raman spectrum.
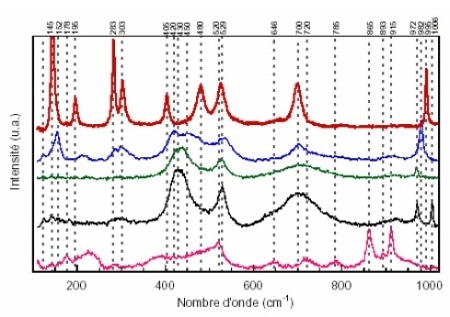
Figure 4. Raman spectra of α, ε, ε', δ,γ phases.(from top to bottom)
When the discharge is performed too rapidly (high current) there are risks for an inhomogeneous intercalation of Li in V2O5 that could lead to short term aging or failure of the battery. Thus these reference spectra are used to monitor the homogeneity of the lithium intercalation process in LixV2O5 grains throughout the cathode during rapid discharge.
As the battery is discharged, spectra are recorded on 25 different V2O5 grains of the cathode. Preliminary results evidenced marked differences among 25 spectra for a given average intercalation rate x, revealing the inhomogeneous intercalation process of Li+ into V2O5.
This may be due to poor electrical contacts between the active material and the current collector and/or to discharge rates that were too high. Improvements of these two aspects in the cell led to similar spectra for the 25 investigated points. Figure 5 plots simultaneously the potential curve and the characteristic Raman intensities of the consecutive LixV2O5 phases. Each plateau shows a phase transition and each drop corresponds to a monophasic domain. Raman results are very well correlated with the potential curve since each plateau see the increase of a new phase at the expense of the previous one. Confocal Raman microspectrometry is hence a complementary probe other than the electrical tests to have a better understanding of the intercalation occurring in the composite electrode.
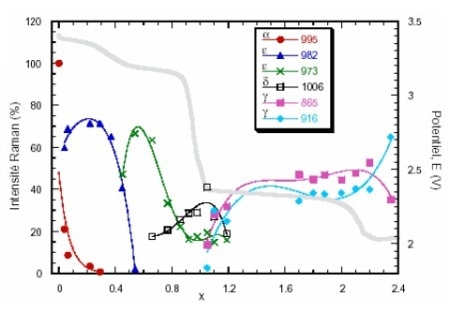
Figure 5. Intensity of the different phases α, ε, δ, γ as a function of the Lithium intercalation rate (x). For each x value, the Raman intensity is averaged over 25 spectra recorded on the electrode.
Summary
Raman enables in situ characterization of microbatteries through an observation window. This will enable correlations between battery performance and molecular/crystallinity changes. Furthermore, the capabilities of current Raman microscopes, which include fast mapping and high throughput to follow the rapid ion diffusion and migration and high spatial resolution to discriminate down to ~1µm3 volumes in the transparent electrolyte.

This information has been sourced, reviewed and adapted from materials provided by HORIBA.
For more information on this source, please visit HORIBA.