Nicomp 380 Dynamic Light Scattering (DLS) Particle Size Analyzer coupled with a multi-angle goniometer can be used to size proteins, prepared by means of hot and cold techniques, at 20, 60 and 90 degrees. This capability in conjunction with a high gain avalanche photo-diode detector (APD) and a high-powered 15mW laser diode can provide a gain increase of 12 compared to the standard Nicomp 380. Low angle DLS could yield key data on the aggregation of proteins and other macromolecules due to the ability of larger particles to scatter relatively more light in the forward direction.
The DLS Technique
DLS is a standard particle sizing technique to size sub-micron particles. The intensity of light scattered in a specific direction by dispersed particles has the tendency to be changed periodically with time. These changes in the intensity versus time profile are due to the continuous changes in the position of the particles caused by Brownian motion.
DLS devices obtain a correlation function from the intensity versus time profile. This exponentially decaying correlation function is investigated for characteristic decay times, which are correlated to particle radius by the Stokes-Einstein equation and then to diffusion coefficients. Compared to laser diffraction, the DLS technique has several advantages. DLS provides an absolute measurement as it does not require knowledge of the composition and optical properties of the suspended particles.
The patented Nicomp analysis algorithm enables measuring skewed unimodal or close bi-modals with a relatively high resolution and accuracy. The DLS technique is capable of accurately sizing particles tinier than 50nm, and determining the size of small protein molecules rapidly and easily. Additionally, multi-angle DLS can be used to acquire quantitative information about protein aggregation.
Short Introduction to the Nicomp DLS System
Analysis with Multi-Angle Nicomp 380
Here, BSA prepared by hot and cold methods was analyzed using the Multi-Angle Nicomp 380 equipped with an APD and a high-powered 15mW laser diode. Figure 1 shows the Intensity-weighted PSDs of each protein acquired at 90° to the incident beam. Each PSD contains two peaks: one at 50nm and the other around 8nm.
.jpg)
Figure 1. DLS Size Distribution of Proteins at 90°. a. Cold Prep; b. Hot Prep.
The first peak represents the native protein. The mean diameter is in line with the earlier measurements taken on the same protein. The secondary peak may represent the aggregates present in the sample and is obscure because the view volume contains only a small number of these particles.
The angle of detection for the Nicomp 380 was lowered to 20° relative to the incident light in order to increase the strength of signal obtained from the aggregate particles. In this manner, it is possible to obtain a more accurate accounting of the aggregates. Figure 2 shows the Intensity-weighted PSDs obtained at 20° for each preparation. Here again, the resulting PSDs comprise two peaks with the native peak at 8nm, but with a strong and well-defined aggregate peak.
.jpg)
Figure 2. DLS Size Distribution of Proteins at 20°. a. Cold Prep; b. Hot Prep.
This data shows the excellent resolution exhibited by the Nicomp 380, both at 90° and 20°, and the instrument’s ability to isolate the native peak from the aggregates in each case. Moreover, the unchanged mean diameter of the native peak at each angle illustrates the instrument’s multi-angle capability.
Figure 3 shows the 20° DLS data from the warm prep sample after filtration, separately, through two different filters, one which filters 100,000 MW unit particles and the other which filtered 1 million MW unit particles. Here, the aggregate peak dominated the PSDs for each filtered sample. Several new aliquots of sample corroborated these results.
.jpg)
Figure 3. DLS at 20° of Warm Prep. After Filtration. a. 100K MWU; b. 1 Meg MWU.
Figure 4 illustrates the results of measurements taken at 20° by passing through an aliquot of puire water through the 100 K filter. Large scattering intensities were identified at 20° and the study yielded a peak at 330nm, which is similar to the mean diameter obtained from the 100 K filtered protein sample.
To know the influence of the filter on the results, an aliquot of water was tested without filtering. Those results were also delineated in Figure 4, showing a peak at 35nm with the largest scattering intensity observed at 20°. The reason behind this is that nanopure systems and other filtering equipment have the tendency to aerate water.
.jpg)
Figure 4. DLS at 20° of water used to dilute proteins. a. Unfiltered; b. Filtered 100K MWU.
Figure 4a shows the presence of microbubbles with a size of 35nm. The bubbles aggregated when the water was filtered, as represented by the results shown in Figure 4b. Sonication is one method of de-aerating water. Light sonication was applied to the warm preperation sample after passing through the 100 K filter. The results are illustrated in Figure 5. Although the aggregate peak can still be seen, the native protein peak could now be observed due to the decrease in the amount of microbubbles. These results are in line with the bubble theory.
.jpg)
Figure 5. DLS at 20°of Hot Prep Protein after Filtering 100K MWU and Sonication.
Conclusion
The results clearly demonstrate the multi-angle capability of the Nicomp 380 and how it can be applied in protein and other macromolecule applications. They also show the potential of the cost-effective 15mW/APD additions.
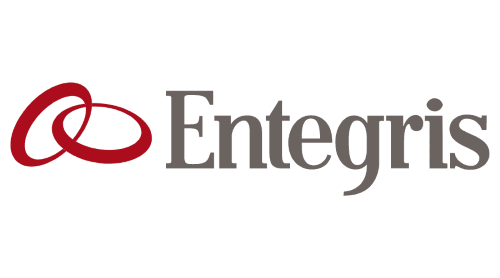
This information has been sourced, reviewed and adapted from materials provided by Entegris
For more information on this source, please visit Entegris