The Gemological Institute of Valenza and Italian researchers from the Department of Earth and Environmental Science at the University of Pavia have published a study on the characteristics of synthetic and natural emeralds using the AvaSpec-ULS2048 Spectrometer for broadband reflectance measurements (400-1000 nm). The team provides their methodology for the classification of these gemstones.
.jpg)
Spectroscopy in Gemology
Spectroscopy was first used for studying minerals and gems soon after Isaac Newton attempted to separate sunlight into its component colors with the help of a prism. By the mid-1800s, researchers began to map the solar spectrum and started to detect absorption spectra for many common elements present in gemstones.
.jpg)
At present, the technology used to produce synthetic gemstones makes it difficult for the gem market to identify treatments applied to stones, identify and classify natural versus synthetic gemstones, and in certain cases, identify the place of origin of a stone.
As a result, the gem market is increasingly employing photonics technologies to meet the demands of the fine jewelry and gem industry.
Natural Emerald Characteristics
A gemstone in the beryl family of minerals, emerald, has a characteristic deep green color because of the presence of iron and chromium. The gemstones are regarded as a type III gem owing to the ubiquitous presence of surface breaking fissures and inclusions.
Due to these surface fissures, emeralds that are commercially available are oil treated with cedar oil which possesses a similar refractive index.
.jpg)
Emeralds can be found worldwide. In fact, they were mined in ancient Egypt circa 1500 BCE and also in Austria and India dating back to the 14th Century. Presently, Brazil, Colombia, and Zambia are some of the largest areas known for emerald production.
Creating Synthetic Emeralds
Emeralds have popular and mystique qualities, which have made this stone one of the most frequently imitated gems. The late 1800s saw the advent of synthetic stones, and in the 1930s the production of the first artificially produced emeralds that were large enough for gem cutting.
Two principle methods are available for producing synthetic emeralds - hydrothermal growth and flux-fusion method. The latter is an old but well-known method used for producing lab-created emeralds. This method uses a flux, or a heated solution of minerals, that forms crystals upon cooling. However, this is a time-consuming method and suitable crystals require approximately one year to grow. The hydrothermal method was introduced in the 1960s and is equally time consuming. It uses pressure and heat to imitate the conditions created in the Earth’s crust.
New and increasingly refined synthetic gems have been introduced since the 1990s, which more closely imitate the physical characteristics of natural emeralds, including the ubiquitous presence of inclusions. This makes reliable classification and identification by reputable gem traders much more significant to the market.
.png)
.png)
.png)
Synthetic Gems in the Marketplace
Synthetic gems are similar to natural counterparts and share almost all of their optical, chemical, and physical characteristics. These artificial stones cannot be distinguished by the naked eye. However, since they can be created to order, large and excellent quality is not as rare among artificial gemstones.
The sale and marketing of synthetic gems is regulated by the Federal Trade Commission and requires disclosure of a gem’s origin and composition. In addition, international gem trade associations and the American Gem Trade Association (AGTA) provide regulations and guidelines to their members about the disclosure of the synthetic origin of a gem at the time of sale.
Using Visible/Near-Infrared Spectroscopy for Identification
The Italian team worked with a selection of 22 synthetic and 19 natural faceted gems. The refractive indexes of the synthetic gems ranged from 1.560 to 1.580 while the natural gems had refractive indexes from 1.571 to 1.598.
As mentioned above, emeralds have a distinctive green color due to the presence of Iron and Chromium. All the samples showed broad absorption bands near 600 nm and 430 nm with peaks at around 683, 680, and 476 nm attributed to chromium ions. Iron contributes typical spectral lines at 465 nm and 810 nm.
.jpg)
By grouping samples by process for synthetic stones and by origin for natural stones, distinct characteristics were shown by the reflectance spectra for each group. However, in combination with other gem analysis techniques, they should enable reliable discrimination of natural from synthetic stones (see figures).
.png)
.png)
.png)
Advantages of Broadband Spectra Analysis
This spectroscopic method provides the biggest advantages for gem analysis — speed of spectra acquisition and ease of sample preparation. At room temperature, broadband spectroscopic analysis can occur on cut stones and the warming effect of the incident light protects the gemstone from thermal cycling which can induce damage. In addition, spectral data can be obtained rapidly and this technique has been tested under usual laboratory conditions.
Noise is present at low wavelengths (400-500 nm), more so among synthetic gems. This can be mitigated by using ultra-low noise spectrometers like the AvaSpec-ULS2048 Spectrometer which offers fast data acquisition (1.1 ms/scan) and excellent signal to noise performance (200:1).
.png)
.png)
.png)
Spectroscopy Applications in Gemology
Spectroscopy is used for identifying synthetic gemstones, as well as for detecting gem treatments or identifying the origin of stones that may come from conflict zones.
Apart from the AvaSpec-ULS2048L spectrometer, a standard configuration for gemological applications comprises of an integrating sphere such as the AvaLight-Hal-Mini halogen lamp light source and fiber optic reflection probe, or the AvaSphere-50-LS-Hal with integrated halogen light source.
Raman spectroscopic measurements are favored by some spectroscopy applications for gemology. Avantes provides its AvaRaman integrated Raman systems for 532 and 785 nm Raman, and also offers a range of thermo-electrically cooled spectrometer options that can be configured for all visible wavelengths.
Sources and Further Reading
- BV, S. B. (2017, February 24). Gemology - Using Spectroscopy for Advanced Gemstone Analysis. Retrieved March 27, 2017, from https://www.azom.com/article.aspx?ArticleID=10513
- Emerald. (2017, March 26). Retrieved March 27, 2017, from https://en.wikipedia.org/wiki/Emerald
- Fritsch, E., & Stockton, C. M. (1987, 04). Infrared Spectroscopy in Gem Identification. Gems & Gemology, 23(1), 18-26. doi:10.5741/gems.23.1.18
- An Introduction to Synthetic Gem Materials. (n.d.). Retrieved March 27, 2017, from https://www.gia.edu/gem-synthetic
- Karampelas, S., Fritsch, E., Zorba, T., & Paraskevopoulos, K. M. (2011, 09). Infrared Spectroscopy of Natural vs. Synthetic Amethyst: An Update. Gems & Gemology, 47(3), 196-201. doi:10.5741/gems.47.3.196
- Martin, F., Mérigoux, H., & Zecchini, P. (1989, 01). Reflectance Infrared Spectroscopy in Gemology. Gems & Gemology, 25(4), 226-231. doi:10.5741/gems.25.4.226
- Stockton, C. M., & Kane, R. E. (1988, 04). The Distinction of Natural from Synthetic Alexandrite by Infrared Spectroscopy. Gems & Gemology, 24(1), 44-46. doi:10.5741/gems.24.1.44
- Stockton, C. M. (1987, 06). The Separation of Natural from Synthetic Emeralds by Infrared Spectroscopy. Gems & Gemology, 23(2), 96-99. doi:10.5741/gems.23.2.96
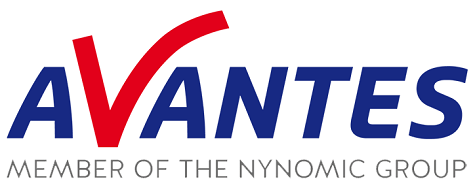
This information has been sourced, reviewed and adapted from materials provided by Avantes BV.
For more information on this source, please visit Avantes BV.