Introduction
Restoring works of art is a challenging task and there is always the risk of causing unintentional damage to the object itself. Paintings on murals, canvas, parchments or papers are particularly vulnerable due to their heterogeneous structure.
It is therefore essential to collect as much information as possible about the nature of the ingredients and the materials involved during restoration work. This includes details about the chemical identity of used components in order to find a suitable cleaning agent, for example, to remove surface impurities or an altered varnish layer from a painting.
Another task is the reproduction of historic paints for scientific reasons. In this case, knowing the chemical nature of a certain paint is key to success. Fourier transform infrared (FTIR) spectroscopy is an ideal technique for the analysis of paints and paintings. Typical absorption patterns in the IR spectrum enable the identification of substances present in the sample.
Until recently, the main disadvantage of this technique was the fact that on-site analyses of artwork were nearly impossible. Measurements on big paintings or murals often demanded destructive sampling as pieces of the sample had to be moved to the laboratory. Of course, in the majority of cases, this is definitely not an option.
Fortunately, non-destructive analysis is easily feasible using the ALPHA II FTIR spectrometer, with its front reflection module. The ALPHA II provides a rapid and easy approach to the infrared analysis of murals and paintings on-site and in a non-destructive and contactless manner.
In the following article, we describe some real-life examples of art analysis by FTIR spectroscopy.
Surface Analysis of a Painting by Mario Schifano
Figure 1. Nottetempo by Mario Schifano with a tripod mounted ALPHA-R in the front.
“Nottetempo” (CSAC - Centro Studi e Archivio della Comunicazione – Parma), a painting created in 1986 by the Italian artist Mario Schifano, is a perfect example to demonstrate that FTIR spectroscopy is capable of analyzing the most complex modern paint compositions. Figure 1 shows the measurement setup with the ALPHA-R being mounted on a tripod in front of the painting.
Figure 2 depicts the raw reflectance spectra measured on two different spots of the painting covered with a blue and white pigment, respectively. At first glance, both spectra look quite complicated and expose artifacts that generally do not appear in infrared spectra.
These effects however, are typical for reflection spectra because of the anomalous dispersion that takes place in spectral regions which show a strong light absorption. Consequently, the bands of the spectra are distorted and look similar to those of a first derivative curve. The Kramers Kronig transformation (KKT) that is incorporated in Bruker’s spectroscopic software OPUS, can be used to convert these distortions into absorption bands.
Figure 2. Raw reflection spectra of two different measuring points with two different pigments (blue pigment = blue spectrum, white pigment = red spectrum).
Figure 3 shows the KKT corrected spectrum of the white pigment as the black curve. It is evident that the spectrum now looks like a “normal” absorption spectrum. Using spectral reference libraries, the sample spectrum's complex mixture of components was identified.
The components were combined in a reference sum spectrum (shown as the red curve in Figure 3). Reference spectra of titanium white pigment, alkidic resin, and nitrocellulose (used as a binder) were used for this calculation. Immediately, the resemblance of both the spectra becomes clear, as the sum spectrum has a fitting counterpart for all bands of the sample spectrum. This indicates that all the key components of the paint have been identified.
Figure 3. KKT transformed sample spectrum of a white pigmented area (black curve) and sum spectrum of different reference spectra (red curve).
The procedure was repeated for the blue pigment, yielding the spectra shown in Figure 4. The black curve represents the KKT transformed spectrum of the pigment. The chemical composition is again evident when the spectrum is compared to the sum spectrum of two reference spectra: Poly(methyl methacrylate:ethyl acrylate), 3:2 and cobalt blue. A striking similarity between both spectra is again observed, implying that all significant constituents of the paint have been established.
This case study reveales the real advantage of this technique: the access to a very broad spectral range. Here, the option to investigate wave numbers down to about 400 cm-1 provides the opportunity to identify even the inorganic pigments next to organic binders or ground layers. Cobalt Blue and Titanium White only show fingerprint signals below 800 cm-1, a region inaccessible to spectrometers using MIR calcogenide fiber optics.
Figure 4. KKT transformed sample spectrum of a blue pigmented area (black curve) and sum spectrum of different reference spectra (red curve).
Surface Analysis of a Painting by Giotto2
These measurements can be used to analyze ancient paintings too. Figure 5 is a highly acclaimed painting by Giotto (Polittico, about 1330, Pinacoteca Nazionale di Bologna, Italy) during the FTIR measurements with a tripod mounted ALPHA spectrometer.
Figure 5. Polittico di Giotto with a tripod mounted ALPHA-R and a laptop PC.
Figure 6 shows the result of the analysis of a region on the wing of the angel located on the second panel from the left (Arcangelo Gabriele). The camera-view of the measuring point is shown in the small inset picture. Here, the ground layer (gold foil) gives a so-called transflectance spectrum, which is directly comparable to an absorbance spectrum without the need for a KKT. Next to the sample spectrum, the reference spectra of calcium oxalate, calcium sulfate, and terpenic varnish are shown. The combination of these three reference spectra accounts clearly for all major absorbance bands in the sample spectrum, which indicates the detection of all essential constituents.3
Figure 6. Detail of panel and video image of the measurement point (left). Right: Sample spectrum (black) and reference spectra of terpenic varnish (blue), calcium sulfate (red) and calcium oxalate (green).
Instrumentation
The operation of ALPHA FTIR spectrometer is straightforward. It is insensitive to shocks and vibrations, enabling operation even in busy environments. Weighing a small 7kg with the footprint of a laboratory notebook, it can be quickly moved to another location and used immediately, since it does not require manual adjustments afterwards. Figure 7 shows the ALPHA II, which features advanced connectivity features, an optional touch PC and further performance enhancements.
The front reflexion module features an integrated video camera, providing a direct and clear visual of the measurement area. Additionally, it enables the user to position the spectrometer exactly at the region of interest. Since the working distance between the object and the ALPHA II is approximately 15 mm, any undesirable accidental contact is safely prevented. The usual sample spot size of about 5mm, can optionally be decreased to 3mm, if higher spatial resolution is required. Moreover, the ALPHA II FTIR spectrometer can be operated cordless by using a Wi-Fi connection and a battery pack.
Figure 7. ALPHA II FTIR spectrometer with front-reflection module.
Summary
The ALPHA FTIR spectrometer with the front reflection module is a flexible and user-friendly instrument to identify all types of materials such as varnishes or pigments. The contactless and non-destructive analysis can be carried out on-site. Bruker´s OPUS software provides an extensive range of powerful tools which are still easy to use for the post-processing and analysis of the measured spectra. To sum up, FTIR spectroscopy can provide essential information on all kinds of paints for both conservation and restoration.
1 In cooperation with D. Bersani, A. Casoli and P. P. Lottici, University of Parma, Italy
2 In cooperation with D. Cauzzi, Soprintendenza BSAE di Bologna, Italy
3 Il Polittico di Giotto nella Pinacoteca di Bologna - Nuove letture, D. Cauzzi e C. Seccaroni, Centro Di (2009)
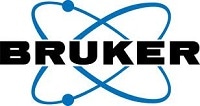
This information has been sourced, reviewed and adapted from materials provided by Bruker Optics.
For more information on this source, please visit Bruker Optics.