When asked about forensics and forensics science, many people could talk about crime scene investigation (CSI), ocular inspection and evidence recovery. These are regularly used terms in modern TV series and films, and have therefore become the sole association in the public consciousness. However, there is much more to forensics than CSI, with the field encompassing various sciences, disciplines and studies such as forensic genetics, chemistry, informatics, anthropology, entomology, ballistics, dactylography, questioned documents, acoustics and linguistics.
With such a large arsenal of tools and techniques to draw from in the process of crime investigation, an investigator might find that where one discipline is crucial in one crime, another might be completely dispensable.[1]
Saying that, there is one discipline in particular that has some use in nearly every case, and usually a lot of use: forensic chemistry. A crime scene[2] will almost always have some substance (either illicit, under suspicion, unknown or even usual daily-life products) that will provide information either on their own, or through a link between it and the persons involved.
This principle, known as Locard’s exchange, states ‘Every contact leaves a trace’ and forms the basis of any forensic investigation.[3]
Wherever somebody steps, whatever somebody touches, whatever somebody leaves, even unconsciously, will serve as a silent witness against him. Not only his fingerprints or his footprints, but his hair, the fibers from his clothes, the glass he breaks, the tool mark he leaves, the paint he scratches, the blood or semen he deposits or collects. All of these and more, bear mute witness against him. This is evidence that does not forget. It is not confused by the excitement of the moment. It is not absent because human witnesses are. It is factual evidence. Physical evidence cannot be wrong, it cannot perjure itself, it cannot be wholly absent. Only human failure to find it, study and understand it, can diminish its value.[4]
Paul L. Kirk, Famed chemist and forensic scientist
This means anything left or altered at the crime scene bears some value in identifying a suspect, be it fingerprints, footprints, tire marks, hair, skin, bodily fluids, textile fibers, glass fragments, tool marks, paints and dyes, explosives, drugs, gunshot residues, remains of inflammable liquids, inks and documents. The majority of evidence in this form is processed and investigated at a forensic chemical laboratory that uses traditional chemical tests or modern analytical techniques to analyze and asses.[1,2]
We are currently in the midst of this switch from the more traditional chemical methods to high-tech modern analysis. Spectroscopic techniques in particular are becoming faster, more sensitive, more compact and portable, and more flexible, which is seeing their usage increase across the forensic field for wider substance analysis.
In tandem with this, spectral libraries are being created that hold a wealth of spectral data for compounds, augmenting this method of analysis. Among these novel spectroscopic techniques is portable Raman spectroscopy. Forensic specialists value Raman spectroscopy as they use it extensively within the laboratory to detect a large number of chemical signatures including those found in explosives, drugs, paints, textile fibers and inks.[5]
However, it was thought that the use of lab-grade Raman spectroscopy in the field would remain in the realms of TV series until up to a few years ago. Several improvements in technology have now meant that portable Raman spectrometers are available for in-situ use, with their performance being comparable to lab-based spectrometers.
This article will present some extraordinarily demanding and challenging applications that would benefit from an in-situ analysis, and relay how the portable spectrometer fared.
Instrumentation
For all of the tests described, an i-Raman® Pro Portable Raman system from B&W Tek (Newark, DE, USA) equipped with a 785 nm-wavelength laser was used. This spectrometer has a powerful detector that requires cooling to -25 °C. This unit can be used in two analysis modes: direct analysis using the Raman probe, and microscopic analysis using an adapted microscope that the Raman probe can easily attach to.
This second mode allows the detection and Raman analysis of microscopic traces that aren’t able to be directly analyzed by the probe. To collect the spectra the BWSpec® software was used that may be controlled using either the inbuilt display or an external laptop. After measurements have been taken, the data can be exported to more powerful spectroscopy and chemometric software for further analysis.
Results
Identification of Pre- and Post-Blast Explosives Particles
The procedure for identifying suspected explosive compounds is vital and dangerous. Once neutralized, the explosive charge of a bomb or improvised explosive device (IED) must be analyzed so that the chemical composition can be confirmed and the main explosive compounds identified.
In this study, a small amount (around 0.2 mg) of a number of organic explosives (TNT, TATP, and PETN) and inorganic oxidizing salts (ammonium nitrate, potassium nitrate and sodium chlorate) used in the fabrication of IEDs were measured using the probe. The different instrumental parameters (laser power, acquisition time and number of scans) were tested for each explosive and inorganic salt. Figure 1 shows some of the spectra of TNT at different laser power and acquisition times. To the naked eye, no differences were found between spectra.
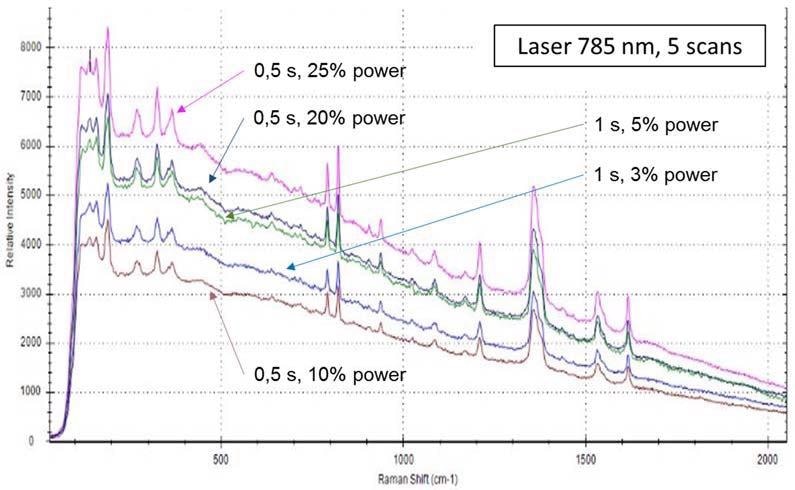
Figure 1. TNT spectra obtained using a 785 nm laser and 5 scans. Different acquisition times and laser power were applied, as shown.
The last step was finalizing the parameters. Those selected for spectrum acquisition were 5 scans, 0.5 s and 10% laser power, using a 785 nm laser, as they have reasonable resolution and use a minimum power, which is always recommended when analyzing high-energetic materials. This is sound advice, as when high power was applied to some of these studied particles they were partially burnt or degraded. Figure 2 shows the analyzed data of the (post-blast) dynamite particles. One of them combusted after using 30% laser power.
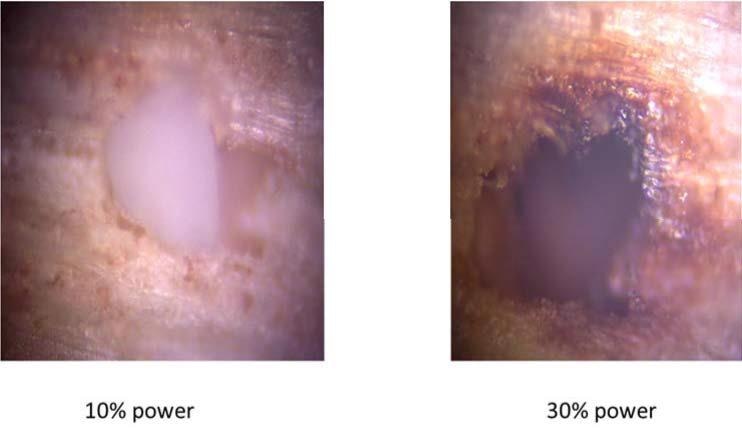
Figure 2. Post-blast dynamite particle after being irradiated with a laser power of 10% (left) and 30% (right).
Once the parameters were optimized, each explosive and salt was analyzed and their spectrum was collected. In order to make a visual comparison easier, some of the spectra were subjected to baseline correction. Figure 3 shows the collected spectra.
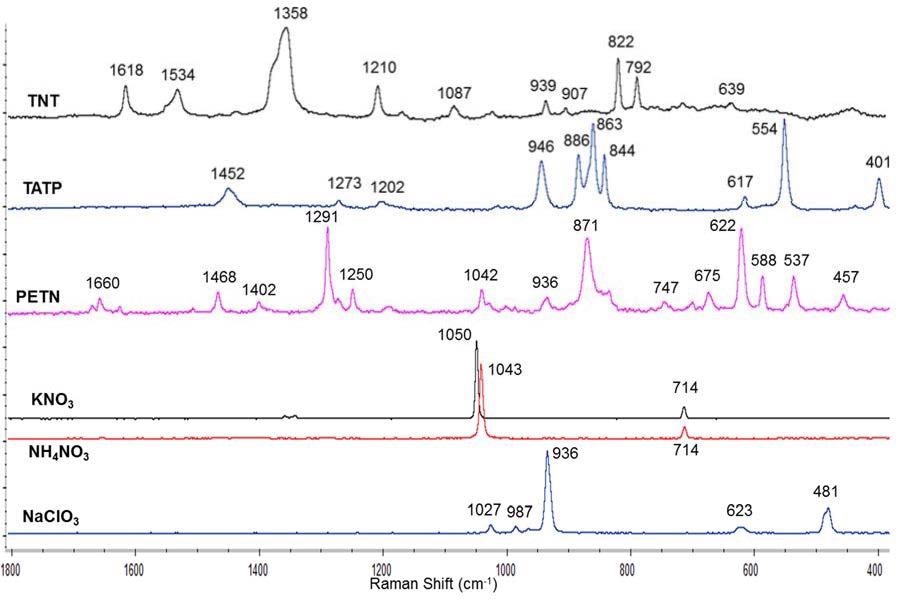
Figure 3. Raman spectra of several explosives and inorganic salts obtained using a 785 nm laser, 5 scans, 0.5 s acquisition time and 10% laser power. Baselines were corrected by software.
The spectra of the explosives and salts were in good agreement with those in the literature and spectra libraries so each substance was confirmed. What is noteworthy is that ammonium nitrate was discriminated from potassium nitrate. If the Raman spectra of these two nitrate salts are compared it can be seen there is a 7 cm-1 difference in the nitrate stretching direction. This difference hadn’t been picked up by the previous handheld and portable spectrometers by the research group, which can be attributed to their limited spatial resolution. This limitation has been overcome by the B&W Tek portable Raman by using a cooled spectrograph at -25 °C.[6]
For a more accurate comparison of explosives and main inorganic salts used in IEDs than a purely visual one, a statistical method in the form of principal component analysis (PCA) using Raman spectra from each explosive was performed.
Figure 4 shows the separated results of the analysis: TNT (black) and PETN (orange) were completely separated from the rest of the explosives, and TATP (brown), potassium nitrate (blue), ammonium nitrate (green) and sodium chlorate (red), though closer, were also separated slightly, so as to make them easier to distinguish.
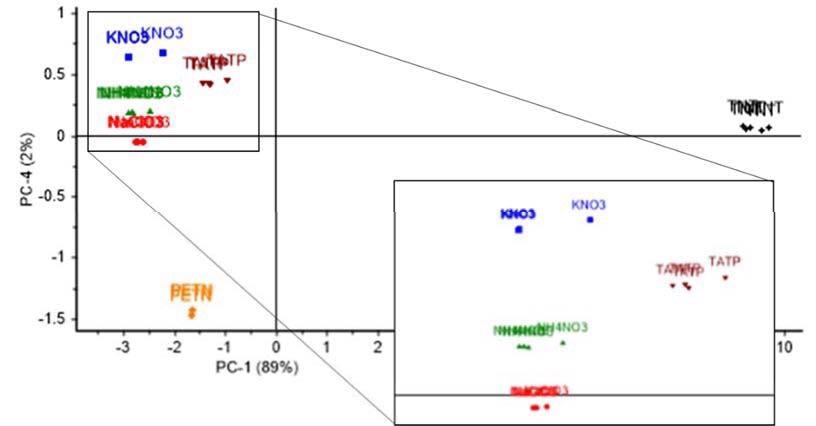
Figure 4. PCA analysis; Scores plot of Principal Component (PC) 1 and 4, showing a variability with a 91% confidence level among all studied intact explosives and inorganic salts: TATP, PETN, KNO3, NH4NO3 and NaClO3.
Similarly, when the explosive material actually explodes, the residues formed by the blast have to be analyzed so that the exact explosive composition can be found. Previous studies have shown a small quantity of unburnt particles from the explosive typically remain spread out all around the crater.
In this study, post blast residues from real explosions using dynamite, ANFO and chloratite were identified and analyzed using the portable microscope coupled to the Raman instrument. This way, even microscopic particles which are undetectable using the probe might be identified using the portable coupled microscope, which is a portable approach of a confocal Raman lab-instrument.
As an example, Figure 5 displays the Raman spectrum of three post-blast particles. Particles of dynamite and ANFO were shown to have the Raman spectrum of ammonium nitrate (the major component in those explosive compositions) whereas the particle of chloratite displayed the Raman spectrum of sodium chlorate (which is the major component in chloratite composition).
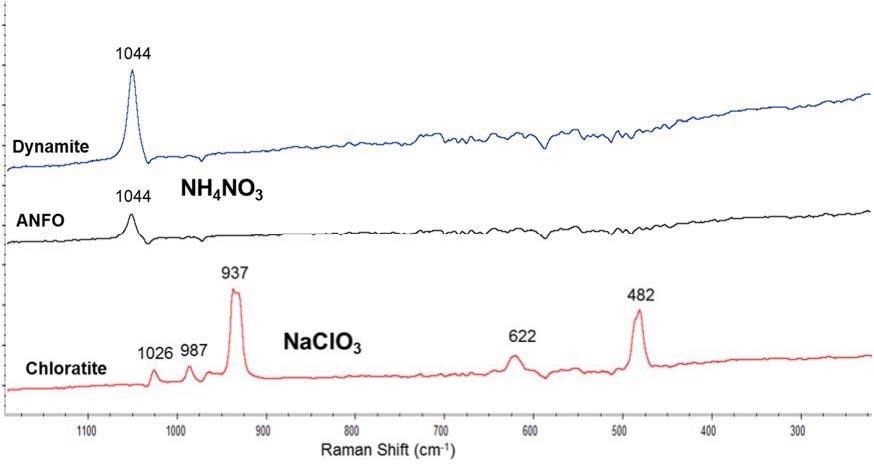
Figure 5. Raman spectra of post-blast particles produced from several explosive compositions. Spectra collected using a 785 nm laser, 5 scans, 0.5 s acquisition time and 10% laser power. Baselines were corrected by software.
Identification of Inflammable Liquids Inside Glass Bottles
Along with IEDs, there is another type of homemade explosive known as the improvised incendiary device (IID). This category of device is designed to cause damage to people, environment, or property by igniting and rapidly spread fire[7] to its immediate area. As with IEDs, they are rough devices made from readily available and cheap components, and usually consist of little more than inflammable liquid, a container, and an ignition source.
Some types may contain hazardous chemicals such as strong acids. The most infamous IID is the Molotov cocktail, which can be found in a more advanced form as the chemical ignition Molotov cocktail (CIMC), made using a closed glass bottle.
For these types of devices it is usually imperative that the suspected contents of the container should not be removed at the scene, so non-invasive identification is vital. This is of interest to first responders or safety officers for security checks at airports or governmental entities.
As well as this on-scene analysis, forensic experts in a lab setting can take advantage of potential non-invasive analysis to avoid the manipulation and exposure of devices such as the CIMC, which may contain hazardous components and by-products (e.g., hydrogen sulfide or sulfuric acid). The measurement of such liquid without opening the container can present a dangerous challenge.
It is fortunate then that the Raman laser penetration is sufficient to reach the liquid in a non-invasive way and obtain the liquid’s Raman spectrum without much interference from the container[7]. With glass bottles, the contribution of the glass is much smaller than that of plastic bottles that can be more overlapping.
This study analyzed four different flammable liquids including: gasoline 98 octane rating, diesel fuel, ethanol and acetone. They were all stored inside glass bottles, and were measured with the probe of the Raman instrument. So as to analyze the largest volume of liquid (i.e. analyzing deeper in the glass bottle) and minimize the contribution from the bottle, the distance regulator at the end of the probe was removed to perform these measurements.
Figure 6 shows the spectra of each liquid. These spectra are in accordance with those in literature and spectral libraries for these inflammable liquids.
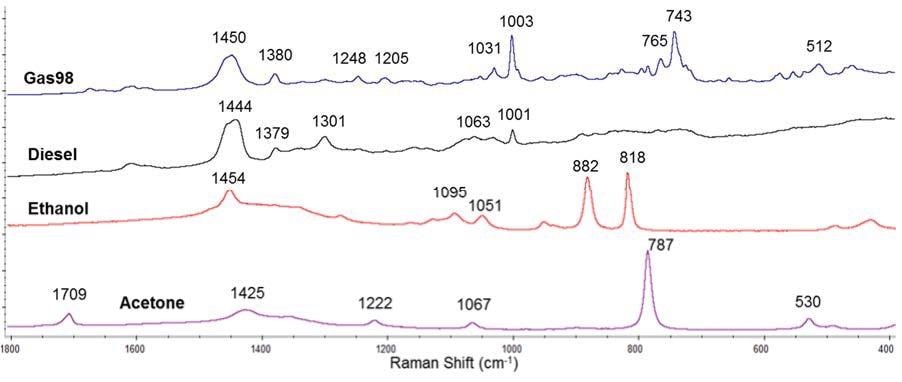
Figure 6. Raman spectra of several inflammable liquids: gasoline 98 octane rating (Gas98), diesel fuel, ethanol and acetone.
A PCA using Raman spectra from each inflammable liquid can be performed to statistically verify their description (Figure 7). As can be seen, those liquids can be clearly discriminated.
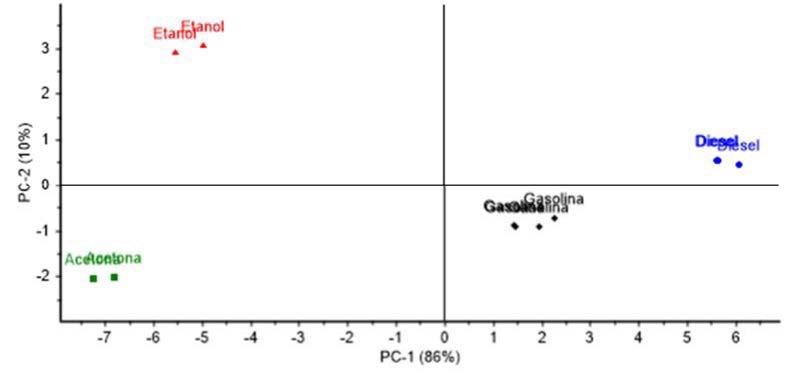
Figure 7. PCA analysis; Scores plot of PC 1 and 2, showing a variability with a 96% confidence level among all studied inflammable liquids: Gasoline 98 octane rating (black diamond marker), diesel fuel (blue circle marker), ethanol (red triangle marker) and acetone (green square marker).
Conclusion
The portable i-Raman Pro has gained more than satisfactory results in the various set challenges. The cooled detector, which greatly improves signal-to-noise ratio and spectral resolution, has proved to be a great advantage and allowed the discrimination of highly similar explosives such as ammonium and potassium nitrate.
In using the microscope attachment, the potential of portable Raman spectrometers is enhanced in such a way that microscopic traces as post-blast explosive particles can be analyzed and identified in-situ. Finally, using the probe through glass bottles can give a rapid and non-invasive in-situ identification of compounds of suspected IIDs. This can improve the safety and decision-making of analyst experts, security forces and first responders.
References and Further Reading
- A.R.W. Jackson, J.M. Jackson, Forensic Science, Pearson Prentice Hall, Harlow, England, 2008.
- L. Kobilinsky, Forensic Chemistry Handbook, Wiley, New Jersey, 2012.
- E. Locard, L’enquête criminelle et les méthodes scientifiques, 1920.
- P. L. Kirk, Crime investigation: physical evidence and the police laboratory. Interscience Publishers, Inc. New York, 1953.
- J. M. Chalmers, H. G. M. Edwards, M. D. Hargreaves, Infrared and Raman spectroscopy in forensic science, Wiley, West Sussex, UK, 2012.
- F. Zapata, C. García-Ruiz, Determination of nanogram microparticles from explosives after real open-air explosions by confocal Raman microscopy, Anal. Chem. 2016, 88, 6726-6733.
- C. Martín-Alberca, M. López-López, C. García-Ruiz, Analysis of pre-ignited Improvised Incendiary Devices using portable Raman, Talanta 2015, 144, 612-618.
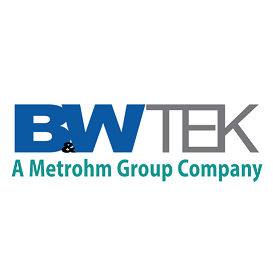
This information has been sourced, reviewed and adapted from materials provided by B&W Tek.
For more information on this source, please visit B&W Tek.