The medical sector continues to experience an increasing interest in implantable devices that are either fully or partially absorbable. Industry leaders are accepting bioabsorbable technology as a means of offering improved patient care in order to realize optimal clinical outcomes. Continual improvements in bioabsorbable polymer technology have enabled clinical developments in this regard.
Absorbable devices, like almost all medical device products, greatly depend on their componentry to improve the effectiveness of the device. Therefore, significant investments continue to be made in the advancement of bioabsorbable polymers as well as the processes required to transform them to device components.
These developments are predominantly focused towards the refinement of polymer properties essential for specialized finished device applications. At this point — component level of the design process — Zeus begins to team up with device manufactures. Zeus thoroughly understands the requirements of device performance and recommends relevant polymer chemistry, morphology, and processing methods designed to realize optimal component functionality.
Performance Factors
The performance of a bioabsorbable medical device component (or finished device) can be classified under four main performance factors — biological, mechanical, dimensional stability, and instability by design (Figure 1).
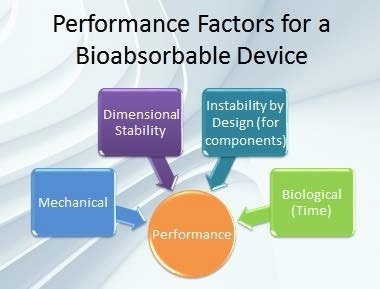
Figure 1. Performance factors for a bioabsorbable device include mechanical factors, dimensional stability, instability by design, and time.
Specific performance criteria defined from these factors offer a framework for component design goals (Figure 2).
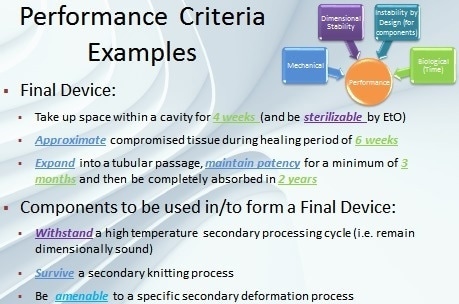
Figure 2. Examples of performance criteria.
Biological – This performance factor covers aspects that range from coatings for drug delivery to load-bearing applications such as vascular scaffolds. When designing the time-dependent properties of a bioabsorbable material, the biological factor of a component or device is very important. The material characteristics of the device should fulfill the biological requirements of the tissue in which it is to be implanted.
Mechanical – These properties always demand considerable attention in device design. For example, properties such as elastic modulus, strength, and elongation-to-break can be crucial in components that may go through conversion process such as knitting or weaving during manufacturing. In addition, mechanical properties are vital for load-bearing devices or devices that will undergo considerable deformation upon implantation or in situ.
Dimensional Stability – Bioabsorbable device components must have a high degree of dimensional stability which is another important performance factor. They must fulfill their ultimate functionality in order to retain their size (and shape). Majority of bioabsorbable devices undergo a terminal sterilization step, for example, which can provide adequate energy to cause dimensional changes if stability is not engineered into the device.
Instability By Design – Usually instability in certain absorbable device components can be beneficial. Several bioabsorbable device manufacturing processes gain from components that have morphologies customized to specific device manufacturing steps such as annealing and heat setting.
Design Controls
The next step after defining the component performance criteria is design. This step encompasses three major design controls: polymer chemistry, polymer processing, and the device geometry. It is possible to match appropriate chemistry with appropriate processing to realize optimal polymer morphology for the device. This synchronization of processing and chemistry is important in component design since properties originating from particular polymer morphologies can be used to help control the geometry of the finished device.
Polymer Chemistry
Consideration of the pertinent polymer chemistry is the prudent place to begin in component design. The chemistry is the fundamental means of governing the absorption profile as well as influencing the mechanical properties of the polymer component. With regards to bioabsorption, the absorption profile is a function of the rate of hydrolysis of the polymer in the body.
This rate of hydrolysis is mediated by the susceptibility to hydrolysis of the ester groups in the polymer chains and by the rate of diffusion of water molecules. For example, when two well-characterized bioabsorbable polymers — polyglycolic acid (PGA) and poly-L-lactic acid (PLLA) — are compared, the rate of hydrolysis of PLLA is found to be much slower than PGA.
The slower hydrolysis rate of PLLA is due to the presence of methyl groups in PLLA’s polymer chains which are absent in PGA. These methyl groups increase the PLLA’s hydrophobicity as well as offer steric hindrance of water molecules which decreases their ability to hydrolyze the nearby ester bonds. Polymer chemistry also has a significant effect on its mechanical properties. The polymer’s ability to crystallize and its glass transition temperature (Tg) are almost completely influenced by the chemistry involved.
Besides PGA and PLLA, there are four other bioabsorbable polymers which are commonly used in FDA-approved devices — polycaprolactone (PCL), poly-DL-lactic acid (PDLLA), poly(trimethylene carbonate) (PTMC), and poly(para-dioxanone) (PPDO) (Table 1). These are specialized polymers that cover a wide range of absorption profiles ranging from weeks to years and also encompass a broad spectrum of mechanical properties from liquid polymers to polymers exhibiting rigidity and high strength.
Copolymerization of monomers offers yet another mechanism to fine tune mechanical properties and absorption profiles; it even allows control over polymer architecture. This level of control enables the creation of synergistic combinations of properties such as toughness and strength that may otherwise be hard to achieve through simpler chemistry.
Table 1. Building blocks of bioabsorbable monomers.
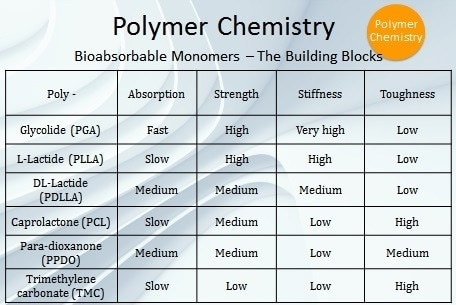
Polymer Processing
Along with polymer chemistry, polymer processing is another indispensable element to consider in the manufacture of bioabsorbable components and devices. Conformance to strict processing guidelines and handling procedures can usually be the difference between success and failure of a product. Similar to polymer chemistry, polymer processing has a major influence on dimensional stability, mechanical properties, and absorption profile of the polymer. As a case in point, almost all bioabsorbable polyesters are melt-processable either through injection molding or extrusion.
At this phase, proper management of degradation during melt processing is critically important. Energy must be added during melt extrusion to mobilize the polymer chains. This same energy can result in degradation during the extrusion process and regeneration of monomer. In addition to this, bioabsorbable polymers are also amenable to a range of solution processes including extrusion casting, electrospinning, or other solvent-based methods.
These aspects show the potential for variability which must be controlled in the polymer processing stage component development and manufacturing.
The effects of polymer processing can be observed by inspecting the conversion of two polymers with considerably different inherent viscosities (IVs) (Figure 3). The materials are both poly lactic-co-glycolic acid (PLGA). The first is from a polymer with an IV of 5.2 dL/g, and the second is from a polymer with an IV of 2.9 dL/g.
The monofilament formed from the 5.2 dL/g IV polymer showed a regenerated monomer level of 2.4% and an IV decrease of 54%. On the other hand, the monofilament produced from the 2.9 dL/g IV polymer showed a considerably lower level of regenerated monomer (0.5%) and a significantly lower decrease in IV (24%). The lower IV polymer, which permitted the use of more optimal processing conditions, was used to achieve reduced polymer degradation during processing.
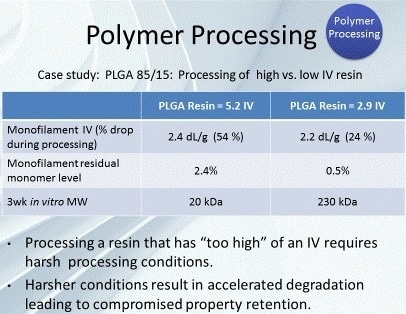
Figure 3. Case study for polymer processing of PLGA 85/15.
In vitro results also showed differences between the two IV polymers (data not shown; see webinar with this same title). Although both monofilaments displayed similar initial tensile strengths, the monofilament from the 5.2 IV polymer exhibited a significantly higher rate of strength loss. After five weeks in vitro, the breaking strength for the monofilament from the 5.2 IV polymer was decreased by 75% whereas the monofilament from the 2.9 IV polymer retained almost 100% of its breaking strength for around 15 weeks.
Polymer Morphology
Although it is basically important to avoid common drawback in polymer processing, specialized processing methods should equally be considered. Actually, these techniques are often highly useful towards achieving optimal product performance. The main advantage of these methods is that they enable more precise control over the polymer morphology, that is, the specific arrangement of the polymer molecules in space. Almost every property of a bioabsorbable polymer is affected by morphology. Morphology is typically categorized on the basis of crystallinity and crystallite or molecular orientation.
While considering crystallinity, two forms are of interest for bioabsorbable devices: amorphous or semi-crystalline. With regards to device manufacturing and processing, chemistry is not the only determinant in crystallite formation. Polymer processing is also an ingredient that affects crystallite formation. Orientation processing is one such example of how processing can be used to direct morphology and polymer properties.
Besides improving mechanical properties such as stiffness and tensile strength, polymer orientation can enhance other material traits such as toughness. This type of processing also impacts absorption profiles through its influence upon crystallite size, crystallinity level, and crystallite orientation from stress-induced crystallization. Therefore, it is the chemistry along with the polymer processing method(s) that eventually allows control of crystalline morphology.
Component/Device Geometry
As mentioned above, processing, morphology, and polymer chemistry all prominently figure into the bioabsorbable component or device design (Figure 4). Interpreting this relationship along with required critical component and device features offers a sound basis for an efficient and effective design. More rational and thoughtful design efforts at the beginning will virtually always lead to lesser design iterations in the future.
As an illustrative example, the design of a polymer vascular scaffold must consider the biological and mechanical performance factors resulting in the crucial performance criteria. Properties such as toughness, a minimum timeframe, and radial strength for those properties to be maintained in the device are but a few of the considerations that should be weighed.
After defining the performance criteria, the next steps that must be considered are appropriate chemistry, morphology, and required processing to bring about that morphology. For example, a morphology exhibiting biaxial orientation with crystallinity calibrated to fulfill the strength and toughness requirements would be an ideal morphology for a vascular scaffold.
Likewise, a well-suited chemistry would be rich in PLLA owing to its ability to crystallize and lock in the molecular and crystallite orientation, and its high glass transition temperature. Furthermore, the absorption profile of PLLA is consistent with the three-month critical mechanical support timeframe. Taking these interconnected variables collectively into account, optimal properties can be attained which will largely impact the sum of the critical geometrical features of the component. A component or device conceived with these considerations will be fundamental to achieving optimal clinical outcomes.
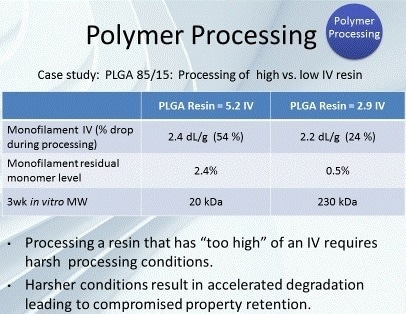
Figure 4. The critical relationship between bioabsorbable chemistry, processing, and device design.
Summary
This article has addressed component-level design controls used to customize bioabsorbable materials and properties required to meet component performance criteria defined by the wider performance requirements of bioabsorbable devices and components. These controls comprise of polymer processing, polymer chemistry, and component geometries as they are linked to the final device geometry.
With regards to material considerations, bioabsorbable polymers are a special group of polymers that need knowledgeable processing, precise handling, and experienced partners who can work with customers to help guarantee proper performance and success of components in the finished device.
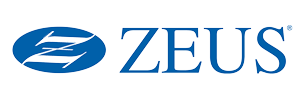
This information has been sourced, reviewed and adapted from materials provided by Zeus Industrial Products, Inc.
For more information on this source, please visit Zeus Industrial Products, Inc.