Sponsored by HORIBAJun 12 2018
Zeta potential is a key physical parameter that describes surface charge on proteins. While measuring the zeta potential of proteins has been a great interest of engineers and scientists for many years, measurement processes oftentimes became challenging due to a variety of reasons.
One of these reasons is the “cooking” of proteins on the cell electrodes when using light scattering techniques. This article presents data from the SZ-100 using cells with unique, patented carbon coated electrodes that facilitate zeta potential measurements of proteins.
Introduction
The surface charge of proteins is a significant physical property that could affect their state aggregation and behavior. A protein’s zeta potential is a useful indicator of stability as well as an effective measure of surface charge. Changes in a protein’s zeta potential could imply any of the following:
- Aggregation
- Conformational changes in protein structure
- Surface modifications
- Unfolding/denaturation of the protein
The zeta potential indicates the degree of repulsion between adjacent, similarly-charged proteins in a dispersion. The principle of general colloid chemistry states that a dispersed system typically loses stability when the magnitude of the zeta potential decreases to less than approximately 30 mV (independent if the charge is positive or negative). As a result, certain regions surrounding the condition would yield zero zeta potential (the isoelectric point, or IEP), making such portion of the system unstable. Within this unstable region, proteins may agglomerate, thereby increasing the measured size. It is, therefore, common application to determine the IEP of a batch of protein sample in analyzing zeta potential.
Although practical considerations with the method of analysis have hindered widespread adoption of such technique, Zeta potential measurements are the preferred way to characterize the charge on the surface of proteins. Classic gold-plated electrodes used in disposable zeta potential cells create Joule-heating that results in the blackening of the electrode surface. This often renders the cell useless after a single measurement. Since the induced electric field is changed by the protein “cooking” onto the electrodes, this heating process not only damages the sample and cell, but also degrades data quality.
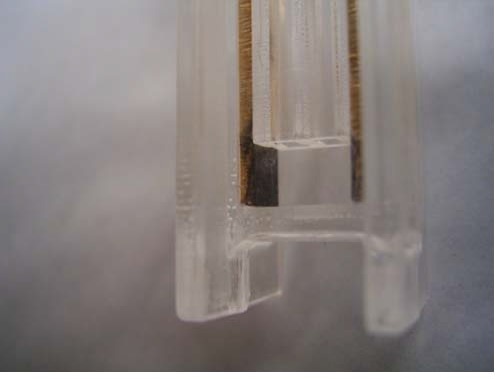
Blackened gold coated electrode surface indicating a ruined cell
HORIBA has pioneered the use of carbon-coated electrodes used in disposable zeta potential cells in order to address measurement challenges of difficult samples, such as proteins. The experiments described in this article were all performed using such method.
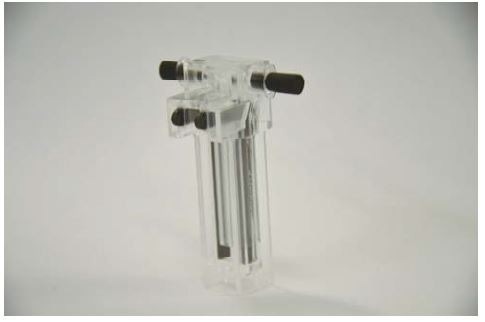
A carbon coated electrode zeta potential cell, usable after several hundred measurements
Experimental
The study utilized proteins that included bovine serum albumin (BSA) and lysozyme. Lyophilized BSA powder acquired from Fisher Scientific (CAS: 9048-46-8) and lyophilized lysozyme powder from Sigma Aldrich (L6876) were prepared by dissolving the powders in ultra-pure DI water at a concentration of 100 mg/mL. Since the pH titration is expected to dilute the sample during the course of the experiment, the concentration level was intentionally increased to a much higher level than the instrument’s sensitivity limit.
Due to the small sample volumes associated with protein work, manual pH titrations were performed instead of utilizing the LY-701 automated titrator. The chemicals used for the pH titrations were 0.1N HCl and 0.1N NaOH. Meanwhile, pH measurements were acquired using a HORIBA TwinpH compact pH meter. An analysis of the zeta potential and particle size measurements were done using the HORIBA SZ-100Z nanoparticle analyzer.
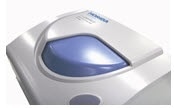
The SZ-100 Nanoparticle Analyzer
Prior to pH titrations, particle size measurements were performed at 90° using a glass cell. The same disposable cell, this time coupled with cell carbon-coated electrodes, was used to measure Zeta potential. The efficiency of the cell in determining results is reliable as it had already been used for over 800 zeta potential measurements on an emulsion sample.
For the lysozyme, the pH was lowered to 4 and then increased at increments of 1 pH unit up to pH 13; meanwhile, for the BSA, the pH was lowered to 3 and then raised up to pH 9.5. Three zeta potential measurements were made at each pH point. The average value was recorded and displayed in the results presented in the next portion.
Results
The particle size of the lysozyme sample was determined to be 4.2 nm prior to beginning the pH titration. The zeta potential results versus. pH values are shown in the below graph. The IEP value of 11.5 is similar to other published results.
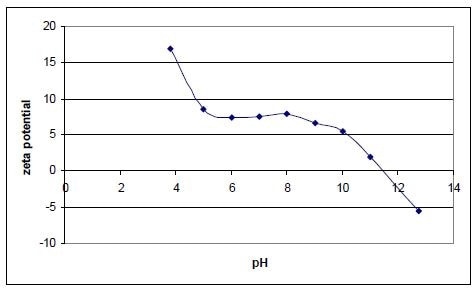
IEP of Lysozyme protein
The particle size of the BSA samples was determined to be 8.4 nm prior to beginning the pH titration. The zeta potential results versus pH values are presented below. The IEP value of 4.6 is similar to other published results.
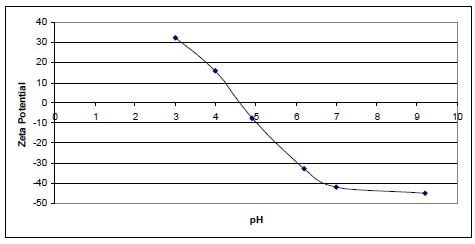
IEP of BSA protein
Conclusions
As hypothesized, the zeta potential of the lysozyme and BSA samples varied as a function of pH. The iso-electric point (IEP) titrations were easily and automatically performed for an estimated one hour each. Meanwhile, thee disposable zeta potential cells with carbon coated electrodes that were used for the measurements presented no signs of degradation. They were later used for other functions without failure.
The innovative use of carbon coated electrodes appears to have transformed the zeta potential analysis of proteins into a simple experimental technique that can be performed by any user; however, salt concentration or other sample properties may risk reducing the lifetime of the zeta potential cell below the expected value. Regardless of such risk, using carbon-coated electrodes promote a wider range of industrial applications and extended cell life.

This information has been sourced, reviewed and adapted from materials provided by HORIBA.
For more information on this source, please visit HORIBA.