Plastics and Polymers
The use of plastics materials has increased dramatically throughout the world over the past 50 years, and now stands at roughly 100 billion pounds.
Polymers
Commercial plastics are known as resins in North America, and are made of polymers. These polymers have been compounded with modifying or stabilizing additives. Typically based on the element carbon, polymer molecules are made from simple, oil-based raw materials. The starting materials for polymers are called monomers and they are small molecules. These small molecules go through a process called polymerization which combines them and forms very large molecules or polymers.
The reason behind the term polymer is due to the final product consisting of several, identical, repeating molecule units. The polymer is sometimes referred to as a ‘high polymer’ or, as a ‘macromolecule’ because usually, the final size (or length) of these molecules and therefore their molecular weight, or mass, is huge.
All plastics are polymers, however, not all polymers are plastics. Several natural products, like cellulose, are polymers, but they are unable to be processed like a commercial plastic material until they are altered from their natural form.
Types of Plastics Material
The definition of a plastic material is a compound of polymer plus additives that has the capabilities of being shaped or molded into a valuable product. This is done in conditions of moderate temperature and pressure. Commonly, plastics have a high stiffness/modulus and a lack of reverse elasticity, allowing them to be distinguished from rubbers or elastomers.
The two key categories of plastic are thermoplastics and thermosetting plastics (thermosets). Thermoplastic products have the ability to be continually softened, melted and reshaped/recycled, for instance in injection molding or extrusion resins. However, thermoset products do not have this property. In terms of tonnage, thermoplastics are undoubtedly the more significant. If the polymer in the plastic is based on one monomer, it uses the term ‘homopolymer.’ If the polymer used is based on two or more monomers, it is referred to as a ‘copolymer.’
.jpg)
Figure 1.
Amorphous and Crystalline
It is possible to further divide thermoplastic materials into two key categories: amorphous and semi-crystalline. An amorphous thermoplastic material has no specific molecular structure and is normally a solid, clear, stiff material with low shrinkage, for example, polystyrene. In comparison, a crystalline polymer has an systematized, “crystal-like” structure, but also comprises of amorphous material.
It is usually known as a semi-crystalline thermoplastic material. These types of plastics are commonly tougher and less fragile compared to amorphous thermoplastics. It is also common for them to have a higher heat distortion temperature.
Additionally, these plastics are translucent, or opaque, and have a high shrinkage and a high specific heat. Polyethylene is the best-known example of a semi-crystalline, thermoplastic material. (Clear polystyrene is sometimes referred to as “crystal polystyrene” and it is an amorphous polymer. However, this name refers to the fact that it is “crystal clear” and not a crystalline thermoplastic.) Both amorphous and semi-crystalline thermoplastics are frequently tested by rheometry and are extensively used in rheological studies.
Names and Abbreviations for Thermoplastics
There are two main types of plastics: thermoplastics and thermosets. Thermoplastics are by far the ones most studied in rheology, therefore, this section will focus only on the major types of thermoplastic material.
.jpg)
Figure 2.
Common Names for Thermoplastics
Most thermoplastics have the prefix ‘poly’, which means ‘many.’ This is followed by the name for the monomer from which the plastic is derived, so the name is source-based. As a result, we get names like polystyrene and polyethylene for homopolymers.
Sometimes the plastic material has more than one word in the name, and when this occurs parentheses may be put around the words, for instance poly (vinyl chloride). This practice is, however, not universal and one may also see the same term without the brackets i.e. polyvinyl chloride.
Other names like ‘acetals’ and ‘cellulosics’ may also be met since source-based nomenclature is not universally used. In addition to this, several plastics are known by more than one name. For instance, acetals may be known as polyformaldehyde or polyoxy-methylene. When this is the case, then the alternative name(s) should also be listed. Common/alternative names of thermoplastics are shown in Table 2.
Abbreviations
Polymers are often referred to by abbreviations because of the complex, chemical names used to describe them. These abbreviations are formed using a short string of capital letters, in which each capital letter denotes a part of the common name. In the case of the plastic material beginning with ‘poly’, the first letter is P and the other letter(s) are taken from the monomer unit. To give an example, polystyrene is shortened to PS and polyethylene is shortened to PE respectively (See Table 2).
On the other hand, copolymers are frequently referred to through initials, in which the monomers used are represented; without a “P” for “poly.” For instance, the copolymer formed from styrene and acrylonitrile is known as styrene acrylonitrile copolymer, or as SAN. When two or more polymers are combined, they are known as blends or alloys, and they are normally represented by the abbreviations used for the individual materials. Each abbreviation is separated by an oblique stroke, such as SAN/EVA.
Relevant Standards
There are several standards organizations that are responsible for issuing standards that specify the letters used when naming plastics. The American Society for Testing and Materials (ASTM) and the International Standards Organization (ISO) are just two that are responsible.
Standard and Non-Standard Abbreviations
It is important to note that both standard and non-standard abbreviations are used. Frequently, materials are formed, and a name is used for them prior to the standards committee suggestions being issued. Consequently, a ‘non standard’ abbreviation may become recognized, or more than one abbreviation may be referred to for the same material. For instance, the thermoplastic elastomer, polyether ester elastomer, is often referred to as PEEL, as COPE (from copolyester), as TEEE (thermoplastic elastomer ether ester) or, as YBPO (an American suggestion). Several abbreviations are given in Table 3.
Thermoplastic Materials
A thermoplastic material has many properties, it softens (or melts) on heating and it hardens (or freezes) on cooling. It is possible for this heating and cooling process to be continually repeated several times. Roughly 80% of all plastics used in this world are thermoplastics making them a very important group of materials that can be divided into four categories (each of which may contain amorphous or crystalline materials):
- Commodity thermoplastics
- Engineering thermoplastics
- Thermoplastic elastomers or rubbers
- Blends or alloys
Commodity Materials
This certain category of plastics covers materials such as polypropylene, polyvinyl chloride, polystyrene and polyethylene (PP, PVC, PS and PE). These materials individually include many sub-divisions. For example, PP contains homopolymers and copolymers, PVC covers stiff and plasticized, PS is used to describe general purpose PS and toughened PS (TPS, or IPS, or HIPS) and PE includes LDPE, LLDPE and HDPE. The importance of commodity thermoplastics cannot be over-emphasized as approximately 80% of all plastics used fall into this category.
Engineering Thermoplastics
Engineering plastics consist of polymers that put forward a mixture of properties, including superior strength, stiffness, robustness and resistance to wear, resistance to chemical attack and heat resistance. There are five major materials included in this group, and they include the polyamides (nylons), acetals, polycarbonates, thermoplastic polyesters and modified polyphenylene oxide (also known as modified polyphenylene ether or PPE).
Thermoplastic Elastomers
Materials referred to as ‘thermoplastic elastomers’ (TPE) or, as ‘thermoplastic rubbers’ (TPR) are a comparatively new group of material. They have become important in relation to both tonnage and value because they are forming new markets in addition to substituting both plastics and traditional rubbers in a number of their applications. They display some properties which are linked with traditional or “vulcanized” rubbers, however, they also can be managed with the haste and effortlessness of thermoplastics, and can be reprocessed.
Blends
There are a number of blends of plastics, or blends of plastics with elastomers due to their ease of production and the fact that they use twin-screw compounding extruders. Moreover, these materials may also be adapted with fillers or glass fiber by blending materials. The reason behind this is because they include the generation of “unusual” plastics materials, lengthening the performance of existing plastics and at a low price whilst also recycling plastic materials.
Property Guidelines
There are several grades of plastics available, so properties that are cited in literature should just be used as an overall guideline, rather than being relied on. This is because the final properties of any plastic material may be largely altered by the processing conditions it is put through and/or by the addition of additives. Variations of basic formulas are accessible using additives in order to provide enhanced heat resistance, or weatherability in many materials.
A number of formulations result in enhanced impact strength, whereas others, which include fillers, are used where moldings should possess the tensile strength and/or high heat distortion temperature, as an example. In addition to this, processing and performance modifiers may also be supplemented. An example of these are lubricants, antistatic additives, and nucleating agents, and these additives may be encompassed as part of a color masterbatch.
Choice Of Grade
After being selected for a particular application, the type of plastic material is considered and the melt viscosity or melt flow rate (MFR or MFI) may be used to further explain the grade to be used. The best choice of grade is dependent on these properties and if, for instance, the components will be subjected to high mechanical stresses, a high viscosity grade may be the best choice.
Having the highest molecular weight and frequently exhibiting superior mechanical properties are just a few reasons why a high viscosity 9 grade would be a good choice. Sometimes, however, this advice should not be followed. An example of this would be the case where undesirable levels of frozen-in strains in the molded part cause a negative result. In this case, an easy flow grade would then be favored, so that it is easier when filling thin injection molded wall sections, or where very smooth surfaces are specified.
Feed Forms
Processing equipment like injection molding machines or extruders, can include plastic resins or compounds in a variety of forms. The feed differs in that it may be a fine powder, regranulated material or pellets. Usually, if uniformly sized pellets are used, higher output rates are obtained. Needless to say, in order to obtain a consistent output, a consistent feed is imperative.
Polyolefins
Probably the best-known example of a semi-crystalline, thermoplastic material is polyethylene. A short discussion of this material is discussed here to describe how this type of material is commonly tested by rheometry and is also largely used in rheology studies.
Polyolefin Materials
Polyethylene (PE) is commonly known as a member of the family of plastics named polyolefins (PO) in which both homopolymer and copolymer polyolefins are obtainable. There are several members of the polyolefin family as seen below.
- Very low density polyethylene (VLDPE)
- Low density polyethylene (LDPE)
- Linear low density polyethylene (LLDPE)
- High density polyethylene (HDPE)
- Metallocene catalyzed polyethylenes (MCPE)
- Polypropylene (PP)
Polyethylene
Polyethylene is made up from a hydrocarbon gas called ethylene and is achieved through the process of polymerization. Described as a simple, low molecular weight (mass) material, ethylene has a molecular weight of 28. When the gaseous ethylene (sometimes called ethene) molecules join together (polymerize) to produce a long chain structure, the molecular size and weight increase dramatically. For example, if ten thousand ethylene molecules are combined together, to result in a film grade of PE, for example, the average molecular weight equals 280,000 atomic units (au).
The polymerization reaction has a random nature, and as a result, a number of the chains will be a different size. These chains will differ in size; some will be larger than the average value and some will be smaller. For instance, some may only contain 20 ethylene units, whereas others may consist of a hundred thousand ethylene units. This results in the molecular weight distribution (MWD) because a statistical distribution of weights (sizes) of the molecules occurs.
Another thing to consider is how the ethylene units are joined together. In a case where two ethylene units add onto another growing chain at the same point, a branching in the chain will occur. Consequently, polyethylene is not essentially a simple long chain material, but is one that may also comprise of many short and long side branches. Material flow and how the molecules are packed together (crystallize) on cooling are affected by these branches.
Material Properties
Polyethylene plastic materials that are available are essentially a family of materials. These may vary in molecular weight, molecular weight distribution and chain branching. Altering any one of these aspects can significantly modify the properties of the material. Subsequently, a wide range of properties for any material group is possible.
The table below shows how changes in melt flow rate (MFR – a measure of molecular weight), density (a measure of molecular packing), and molecular weight distribution (MWD) affect the properties of HDPE.
Effect of Structural Changes for HDPE
Changes in molecular weight, density and molecular weight distribution (MWD) can have many effects and can be seen in the table below.
What are the Different Types of Polyethylene?
Density of PE can be categorized in the following ways:
- Low density of PE (0.910 to 0.925 g/cm³) – sometimes referred to as Type I.
- Medium density of PE (0.926 to 0.94 g/cm³) – sometimes referred to as Type II.
- High density of PE (0.940 to 0.959 g/cm³) – sometimes referred to as Type III.
- Very high density of PE (>0.959 g/cm³) – sometimes referred to as Type IV.
The grades of HDPE with a density below 0.96 are formed by using a second monomer at low levels (<1%). In other words therefore, they are copolymers of PE with another olefin. (For example, with butene –1 or, with hexene –1.) By using the second monomer, it reduces the density by introducing short side chain branches that disturb the crystalline structure. These materials may be called MDPE. In blow molding, HDPE/MDPE copolymers account for approximately 55% of all plastics.
Property |
Effect of Increasing: |
|
MFR |
Density |
Breadth of MWD |
Pseudoplasticity |
|
|
Increases |
Tensile strength at yield |
|
Increases |
|
Tensile strength at break |
Decreases |
Increases |
|
Elongation at break |
Decreases |
Decreases |
|
Impact strength |
Decreases |
Slight decrease |
Decreases |
Modulus |
|
Increases |
|
Transparency |
|
Decreases |
|
Long term load bearing |
|
Increases |
|
ESC resistance |
Decreases |
Decreases |
|
Softening temperature |
|
Increases |
|
Melt strength |
Decreases |
|
Increases |
Melt elasticity |
Decreases |
|
Increases |
Melt fracture tendency |
Decreases |
|
Decreases |
Gloss |
|
|
Decreases |
Property Differences for Polyethylenes
(Compared to the one left blank.)
Property |
Polymer Density in g/cm³ |
|
Low
(Type I) |
Medium
(Type II) |
High
(Type III) |
Tensile strength at rupture |
Highest |
Higher |
|
Elongation at break |
Highest |
Higher |
|
Impact strength |
Highest |
Higher |
|
Modulus |
Highest |
Higher |
|
Transparancy |
Highest |
Higher |
|
Long term load bearing |
|
Higher |
Highest |
ESC resistance |
Highest |
Higher |
|
Softening temperature |
|
Higher |
Highest |
Melt strength |
|
Higher |
Highest |
Gloss |
Highest |
Higher |
|
Resistance to shrinkage |
Highest |
Higher |
|
Resistance to warpage |
Highest |
Higher |
|
Resistance to brittleness at
low temperature |
Highest |
Higher |
|
Resistance to grease and oil
absorption |
|
Higher |
Highest |
Impermiability to gases and
liquids |
|
Higher |
Highest |
Freedom from film haze |
|
Highest |
Higher |
Molding cycle times |
|
Higher |
Highest |
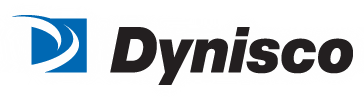
This information has been sourced, reviewed and adapted from materials provided by Dynisco.
For more information on this source, please visit Dynisco.