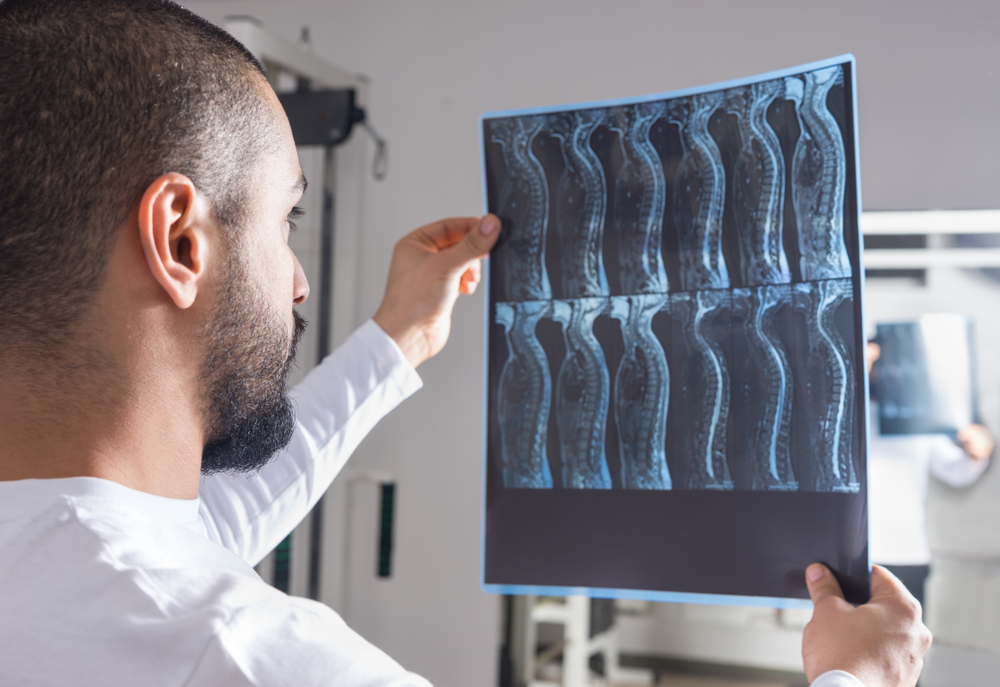
Image Credit: Viacheslav Nikolaenko/Shutterstock.com
Following spinal cord injuries, patients may suffer from partial or even total paralysis. The damage to the central nervous system impairs the brain's ability to send electrical signals to the muscles, limiting motor function significantly. To remedy this, biomedical engineering uses Neuroprostheses, which are applied to the brain, spine, or muscles that send electronic signals to induce movements alongside the damaged nervous system.
Any technology intended to be bio-integrated shares a significant issue of balancing resilience and elasticity. Biological tissue in the nervous system and muscles are capable of elastic deformation (changing shape under stress, with no permanent deformation). The brain is also compiled of very soft organic tissue and will deform under small stresses. For the prosthetic to integrate successfully, it must be tough under stress, but not rigid, and match the tissue's elasticity.
To add to the situation's complexity even further, the organic tissue varies from patient to patient. Age, lifestyle, and past injuries all contribute to differences in the mechanical properties of the tissue. Because of this, it is challenging to make a standard invasive implant that integrates well in all patients due to these variations of each patient’s body.
To combat this, researchers at St Petersburg University have developed a method to 3D print these implants so that they can be easily customized to suit the requirements of the specific patient.
The Printing Method Process
Firstly, plasma activation improves the wettability of the array. This step is necessary to enhance the application of coatings. Plasma activation makes use of “partially ionized gas” (Thomas, S., et al., 2019), inducing polar groups to enhance the surface's ability to adhere to coatings.
The technology then uses extrusion of a “shear thinning silicone elastomer” (Afanasenkau, D., et al., 2020) to create the electrode array's insulating matrix. This extrusion geometry dictates the general shape of the electrode array and its “positions of tissue contact sites”, both of which are customizable aspects of the array to suit the patient’s needs.
Platinum microparticle inks, which are suspended in a viscous solvent, are used (Afanasenkau, D., et al., 2020). This can, like the silicone insulation, be customized depending on the patient. This is then applied to the insulating extrusion via an inkjet.
The evaporation of the solvent will leave platinum sand in the shape of the chosen electrical conductor. Guides for electrical wires, which will later be applied by hand, and conductive paste are also added by extrusion to connect the contact pads to external electronics.
To complete the interface, a low-viscosity silicone seal is applied over the geometry of the array. The low viscosity allows for the silicone to penetrate between the interparticle spaces of the previously applied platinum sand. Though this step does not necessarily improve the interface's customizability, it is an improvement on the speed and ease of manufacture compared to previous processes, which required the conductive and elastomer phase to be mixed to a paste before application.
The completed array is then polymerized thermally and removed from the printing substrate by hand. The final interface is challenging and has significantly high compliance, exceeding a tensile strain of 100% before mechanical failure. This leaves it more than flexible enough for its purpose, given most biological tissue in the nervous system “rarely exceed 20%, beyond which tissue damage may occur” (Afanasenkau, D., et al., 2020).
On the Market Today: 3D Printers and 3D Printing Equipment, Materials and Accessories
Experimental Data on Animals
The NeuroPrint technology has been proven to be efficient in experiments.
The first experiment used electrical spinal cord stimulation (SCS) - a rehabilitation method in which the lumbosacral spinal cord is excited to induce movement in the body. Decerebration refers to removing the cerebrum, the section of the brain responsible for motor function engagement and coordination. The NeuroPrint used continuous SCS to induce a consistent and coordinated walk cycle.
Experiments on zebrafish saw a variation of the NeuroPrint connected to the dorsal fins. The Neuroprint electrodes had a custom geometry to fit the animal, highlighting the technology adaptability. The electrodes were able to record electromyography of the fins during the zebrafishes’ swimming cycle.
Finally, another variation on the neuroprosthetic was tested on a group of rats to ensure that continued use of the devices over long periods did not cause any adverse effects.
The experiment involved implementing spinal electrode arrays to locations in the rats that would endure the most mechanical loading cycles. Other rats underwent the same surgical procedure but did not have the NeuroPrint added. These rats would serve as a control group of the experiment.
After eight weeks, the behavior and microanatomy of the rats were analyzed. Test results revealed no significant difference to either metric between the rats with the NueroPrint, and those without. Implying long-term usage of the device did not cause any unwanted side-effects. As this experiment was done on an area with maximum mechanical loading, it was safe to assume that other implementations of the NeuroPrint would also be safe.
References and Further Reading
Bayly, P.V., et al. (2005) Deformation of the Human Brain Induced by Mild Acceleration. Journal of Neurotrauma. https://www.ncbi.nlm.nih.gov/pmc/articles/PMC2377024/
Afanasenkau, D., et al. (2020) Rapid Prototyping of Soft Bioelectronic Implants for Use as Neuromuscular Interfaces. Nature Biomedical Engineering. https://doi.org/10.1038/s41551-020-00615-7
Ogorodnikova, P. (2020) Technology for Printing Customized Neuroprostheses on a 3D Bioprinter. [Online] EurekAlert. Available at: https://www.eurekalert.org/pub_releases/2020-09/spsu-tfp092320.php (Accessed on 10th October 2020)
SpecialChem. (2020) New 3DP Neuroprosthesis Implant to Restore Motor Functions in Paralyzed Patients. [Online] Omnexus. Available at: https://omnexus.specialchem.com/news/industry-news/new-3dp-neuroprosthesis-implant (Accessed on 10th October 2020)
Thomas, S., et al. (2019) Non-Thermal Plasma Technology for Polymeric Materials. Elsevier Inc., Amsterdam.
Gerasimenko, Y., et al. (2015) Transcutaneous electrical spinal-cord stimulation in humans. Annals of Physical and Rehabilitation Medicine. https://doi.org/10.1016/j.rehab.2015.05.003
Disclaimer: The views expressed here are those of the author expressed in their private capacity and do not necessarily represent the views of AZoM.com Limited T/A AZoNetwork the owner and operator of this website. This disclaimer forms part of the Terms and conditions of use of this website.