Sponsored by MerckJan 4 2021
Graphene oxide (GO, product number 763705) is an exceptional material observed as a solitary monomolecular layer of graphite with numerous oxygen encompassing functionalities like epoxide, carbonyl, carboxyl and hydroxyl groups.1,2,3
Awareness about GO grew hugely following graphene, a single layer of graphite, first being isolated and studied.4 It was originally hoped that GO might be a synthetic precursor to graphene.5 When the electrically insulating GO is lessened, the concentrated graphene oxide (GO, product number 777684) formed bears a resemblance to graphene but holds residual oxygen and additional heteroatoms in addition to structural imperfections.6,7
A production process for rGO that is more resemblant of a pristine graphene is an incredible test in the field. However, because rGO can be created as a thin film from an aqueous dispersion of GO in water and has reasonable conductivity, it is good for application in electronic devices.8,9,10,11,12 Further to being components in electronic devices, GO and rGO have been employed in nanocomposite materials,13,14 polymer composite materials,13 energy storage,14 biomedical applications,15,16,17 catalysis18,19,20 and as a surfactant21 with some intersections across these areas.
Synthesis and Reduction of GO
A lot of current techniques for the synthesis of GO are founded on the technique originally described by Hummers, where graphite is oxidized by a solution of potassium permanganate in sulfuric acid.22 Decreasing GO by employing hydrazine is described.5 Despite this, hydrazine is extremely toxic and can possibly functionalize the GO with nitrogen heteroatoms.23 Due to these problems, substitutes to hydrazine, including NaBH4,14 ascorbic acid,24 and HI25,26 and others have been employed for the lessening of GO. GO can be lessened as a thin film or in an aqueous solution. Reduction techniques were recently appraised.6
Electronics
Many electronic devices were created employing GO as a primary material for one of the components at a minimum. One of these devices is a graphene founded field effect transistor (GFET).27,28 Field effect transistors (FETs) that use rGO have been employed as chemical sensors29,30,31 and biosensors. An illustration of GFET sensors can be seen in Figure 1.31 GFETs employing functionalized rGO as the semiconductor are sometimes utilized as biosensors to spot hormonal catecholamine molecules,32 avidin33 and DNA.34 In a further investigation, GO that was functionalized with glucose oxidase and placed on an electrode was employed as an electrochemical glucose sensor.35
Visible light transparent electrodes are critical for both light emitting diodes (LEDs) and solar cell devices. Because GO may be handled in solution, employing rGO as a transparent electrode is a suitable substitute for other transparent electrodes like ITO for such devices.36,37 As well as being a transparent electrode, rGO has been employed as a hole transport layer in polymer solar cells and LEDs.38,39
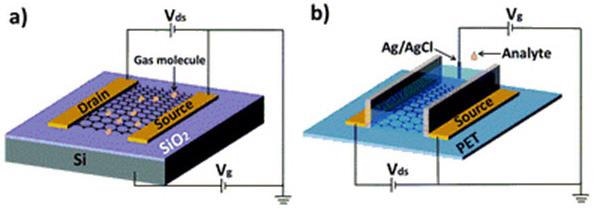
Figure 1. (a) Typical back-gate GFET on Si/SiO2 substrate used as gas sensor. (b) Typical solution-gate GFET on flexible polyethylene terephthalate (PET) substrate used as chemical and biological sensor in aqueous solution. Reproduced from reference 31 with permission from the Centre National de la Recherche Scientifique (CNRS) and The Royal Society of Chemistry.
Energy Storage
Nanocomposites of rGO have been employed for high-capacity energy storage in lithium-ion batteries. In these investigations, electrically insulating metal oxide nanoparticles were adsorbed onto rGO to grow the performance of the materials in batteries.40,41,42,43,44 For instance, the energy storage capability and cycle constancy was proven to rise for Fe3O4 on rGO versus unadulterated Fe3O4 or Fe2O3 (Figure 2).43 Large surface area rGO has been created, employing microwaves for exfoliation and lessening of GO. The large surface area rGO created is beneficial as an energy storing material in supercapacitors.45,46
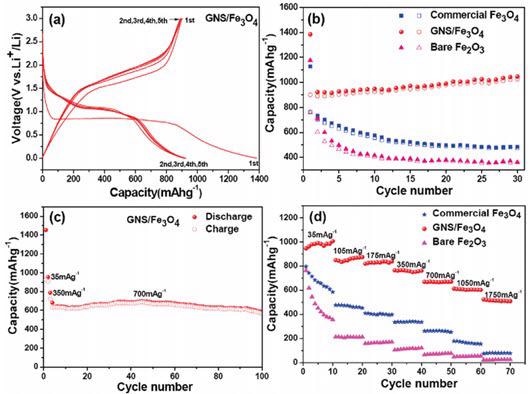
Figure 2. (a) Discharge/charge profiles of the GNS/Fe3O4 composite. (b) Cycling performance of the commercial Fe3O4 particles, GNS/Fe3O4 composite and bare Fe2O3 particles at a current density of 35 mA/g Solid symbols, discharge; hollow symbols, charge. (c) Cycling performance of the GNS/Fe3O4 composite at a current density of 700 mA/g for 100 cycles. (d) Rate performance of the commercial Fe3O4 particles, GNS/Fe3O4 composite, and bare Fe2O3 particles at different current densities. GNS = rGO. Adapted with permission from reference 43. Copyright 2010 American Chemical Society.
Biomedical Applications
One application of GO in the biomedical field is as an element in drug delivery structures. Functionalized nanographene oxide (nGO, product number 795534) is employed in numerous investigations about targeted distribution of anti-cancer drugs. Polyethylene glycol (PEG) functionalized nGO with SN38, a camptothecin (product number H0165) derivative, adsorbed onto the surface (nGO‒PEG‒SN38) was employed as a water and serum soluble foundation of the drug.47 This study, nGO‒PEG‒SN38 was revealed to be three orders of magnitude more impactful than irinotecan (CPT-11), an FDA accepted SN38 prodrug, at decreasing the cell capability of human colon cancer cell lines HTC-116 (Figure 3).47
The success of nGO‒PEG‒SN38 was comparable to SN38 in DMSO as displayed in Figure 3.47 Melanoma skin cancer in mice was treated applying photothermal ablation therapy with a near-infrared laser and nGO that was functionalized with PEG and hyaluronic acid and distributed transdermally.48 Magnetite was adsorbed onto GO loaded with the anti-cancer drug doxorubicin hydrochloride (DXR, product numbers D1515 and 44583) in a different study for targeted distribution of the drug to certain sites employing magnets.49
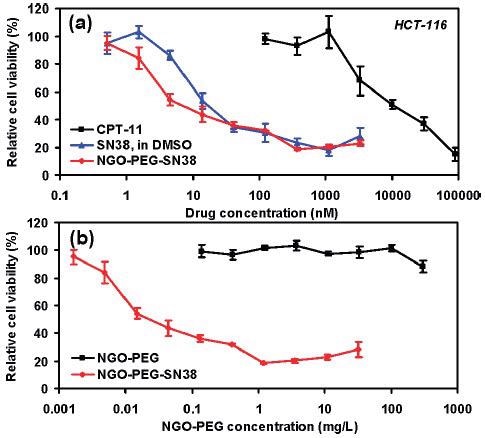
Figure 3. In vitro cell toxicity assay. (a) Relative cell viability (versus untreated control) data of HCT-116 cells incubated with CPT-11, SN38, and NGO‒PEG‒SN38 at different concentrations for 72 h. Free SN38 was dissolved in DMSO and diluted in PBS. Water soluble NGO‒PEG‒SN38 showed similar toxicity as SN38 in DMSO and far higher potency than CPT-11. (b) Relative cell viability data of HCT-116 cells after incubation with nGO-PEG with (red) and without (black) SN38 loading. Plain NGO‒PEG exhibited no obvious toxicity even at very high concentrations. Error bars were based on triplet samples. NGO = nGO. Adapted with permission from reference 47. Copyright 2008 American Chemical Society.
Biosensors
GO and rGO have been employed as parts of numerous systems created to find biologically appropriate molecules. GO has been employed as a fluorescence quenching material in biosensors that apply the fluorescence resonance energy transfer (FRET) effect. In a study by Lu et al., single-stranded DNA (ssDNA) with a fluorescence tag was discovered to tie noncovalently to GO following quenching of the fluorescence of the tag.50 Adding a complementary ssDNA detached the tagged DNA from the GO surface and reinstated the fluorescence. The FRET impact was additionally employed with fluorescein categorized ATP aptamer to sense ATP as low as 10 μM.51 Folic acid-functionalized GO was employed as an element in a system employed to sense human cervical cancer and human breast cancer cells.52
MiliporeSigma provides good quality graphene oxide products for state-of-the-art and progressive materials study requirements.
Acknowledgments
Produced from materials originally authored by Avery Luedtke, Ph.D., from MiliporeSigma
References and Further Reading
- Compton, O. C.; Nguyen, S. T. Graphene Oxide, Highly Reduced Graphene Oxide, and Graphene: Versatile Building Blocks for Carbon-Based Material Small 2010, 6, 711.
- Mao, S.; Pu, H.; Chen, J. Graphene Oxide and its Reduction: Modeling and Experimental Progress RSC Adv., 2012, 2, 2643.
- Dreyer, D. R.; Park, S.; Bielawski, C. W.; Ruoff, R. S. The Chemistry of Graphene Oxide Chem. Soc. Rev. 2010, 39, 228.
- Novoselov, K. S.; Geim, A. K.; Morozov, S. V.; Jiang, D.; Zhang, Y.; Dubonos, S. V.; Grigorieva, I. V.; Firsov, A. A. Electric Field Effect in Atomically Thin Carbon Films Science 2004, 306, 666.
- Gilje, S.; Han, W.; Wang, M.; Wang, K. L.; Kaner, R. B. A Chemical Route to Graphene for Device Applications Nano Lett. 2007, 7, 3394.
- Chua, C. K.; Pumera, M. Chemical Reduction of Graphene Oxide: a Synthetic Chemistry Viewpoint Chem. Soc. Rev. 2014, 43, 291.
- Pei, S.; Cheng, H-M. The Reduction of Graphene Oxide Carbon 2012, 50, 3210.
- Zhu, Y.; Murali, S.; Cai, W.; Li, X.; Suk, J. W.; Potts, J. R.; Ruoff, R. S. Graphene and Graphene Oxide: Synthesis, Properties, and Applications Adv. Mater. 2010, 22, 3906.
- Chen, D.; Feng, H.; Li, J. Graphene Oxide: Preparation, Functionalization, and Electrochemical Applications Chem. Rev. 2012, 112, 6027.
- Wan, X.; Huang, Y.; Chen, Y. Focusing on Energy and Optoelectronic Applications: A Journey for Graphene and Graphene Oxide at Large Scale Acc. Chem. Res. 2012, 45, 598.
- Eda, G.; Chhowalla, M. Chemically Derived Graphene Oxide: Towards Large-Area Thin-Film Electronics and Optoelectronics Adv. Mater. 2010, 22, 2392.
- Wan, X.; Long, G.; Huang, L.; Chen, Y. Graphene – A Promising Material for Organic Photovoltaic Cells Adv. Mater. 2011, 23, 5342.
- Huang, X.; Qi, X.; Boey, F.; Zhang, H. Graphene-Based Composites Chem. Soc. Rev. 2012, 41, 666.
- Lightcap, I.; Kamat, P. V. Graphitic Design: Prospects of Graphene-Based Nanocomposites for Solar Energy Conversion, Storage, and Sensing Acc. Chem. Res. 2013, 46, 2235.
- Sun, X.; Liu, Z.; Welsher, K.; Robinson, J. T.; Goodwin, A.; Zaric, S.; Dai, H. Nano-Graphene Oxide for Cellular Imaging and Drug Delivery Nano. Res. 2008, 1, 203.
- Chung, C.; Kim, Y-K.; Shin, D.; Ryoo, S-R.; Hong, B. H. Min, D-H. Biomedical Applications of Graphene and Graphene Oxide Acc. Chem. Res. 2013, 46, 2211.
- Wang, Y.; Li, Z.; Wang, J.; Li, J.; Lin, Y. Graphene and Graphene Oxide: Biofunctionalization and Applications in Biotechnology Trends Biotechnol. 2011, 29, 205.
- Pyun, J. Graphene Oxide as Catalyst: Application of Carbon Materials Beyond Nanotechnology Angew. Chem. Int. Ed. 2011, 50, 46.
- Yeh, T-F.; Syu, J-M.; Cheng, C.; Chang, T-H.; Teng, H. Graphite Oxide as a Photocatalyst for Hydrogen Production from Water Adv. Funct. Mater. 2010, 20, 2255.
- Dreyer, D. R.; Jia, H-P.; Bielawski, C. W. Graphene Oxide: A Convenient Carbocatalyst for Facilitating Oxidation and Hydration Reactions Angew. Chem. Int. Ed. 2010, 49, 6813.
- Kim, J.; Cote, L. J.; Franklin Kim, F.; Yuan, W.; Shull, K. R.; Huang, J. Graphene Oxide Sheets at Interfaces J. Am. Chem. Soc. 2010, 132, 8180.
- Hummers, W. S.; Offeman, R. E. Preparation of Graphitic Oxide J. Am. Chem. Soc. 1958, 80, 1339.
- Shin,H-J.; Kim, K. K.; Benayad, A.; Yoon, S-M; Park, H. K.; Jung, I-S; Jin, M. H.; Jeong, H-K; Kim, J. M.; Choi, J-Y.; Lee, Y. H. Efficient Reduction of Graphite Oxide by Sodium Borohydride and Its Effect on Electrical Conductance Adv. Funct. Mater. 2009, 19, 1987.
- Fernández-Merino, M. J.; Guardia, L.; Paredes, J. I.; Villar-Rodil, S.; Solís-Fernández, P.; Martínez-Alonso, A.; Tascón, J. M. D. Vitamin C Is an Ideal Substitute for Hydrazine in the Reduction of Graphene Oxide Suspensions J. Phys. Chem. C 2010, 114, 6426.
- Moon, K.; Lee, J.; Ruoff, R.S.; Lee, H. Reduced Graphene Oxide by Chemical Graphitization Nat. Commun. 2010, 1, 73.
- Pei, S.; Zhao, J.; Du, J.; Ren, W.; Cheng, H-M. Direct Reduction of Graphene Oxide Films into Highly Conductive and Flexible Graphene Films by Hydrohalic Acids Carbon 2010, 48, 4466.
- Su, C-Y.; Xu, Y.; Zhang, W.; Zhao, J.; Liu, A.; Tang, X.; Tsai, C-H.; Huang, Y.; Li, L-J. Highly Efficient Restoration of Graphitic Structure in Graphene Oxide Using Alcohol Vapors ACS Nano 2010, 4, 5285.
- Wang , S.; Ang, P. K.; Wang, Z.; Tang, A. L. L.; Thong, J. T. L.; Loh, K. P. High Mobility, Printable, and Solution-Processed Graphene Electronics Nano Lett. 2010, 10, 92.
- Lu, G.; Park, S.; Yu, K.; Ruoff, R. S.; Ocola, L. E.; Rosenmann, D.; Chen, J. Toward Practical Gas Sensing with Highly Reduced Graphene Oxide: A New Signal Processing Method To Circumvent Run-to-Run and Device-to-Device Variations ACS Nano 2011, 5, 1154.
- Chen, K.; Lu, G.; Chang, J.; Mao, S.; Yu, K.; Cui, S.; Chen, J. Hg(II) Ion Detection Using Thermally Reduced Graphene Oxide Decorated with Functionalized Gold Nanoparticles Anal. Chem. 2012, 84, 4057.
- He, Q.; Wu, S.; Yin, Z.; Zhang, H. Graphene-Based Electronic Sensors Chem. Sci. 2012, 3, 1764.
- He, Q.; Sudibya, H. G.; Yin, Z.; Wu, S.; Li, H.; Boey, F.; Huang, W.; Chen, P.; Zhang, H. Centimeter-Long and Large-Scale Micropatterns of Reduced Graphene Oxide Films: Fabrication and Sensing Applications ACS Nano 2010, 4, 3201.
- He, Q.; Wu, S.; Gao, S.; Cao, X.; Yin, Z.; Li, H.; Chen, P.; Zhang, H. Transparent, Flexible, All-Reduced Graphene Oxide Thin Film Transistors ACS Nano 2011, 5, 5038.
- Cai, B.; Wang, S.; Huang, L.; Ning, Y.; Zhang, Z.; Zhang, G-J. Ultrasensitive Label-Free Detection of PNA–DNA Hybridization by Reduced Graphene Oxide Field-Effect Transistor Biosensor ACS Nano 2014, 8, 2632.
- Liu, Y.; Yu, D.; Zeng, C.; Miao, Z.; Dai, L. Biocompatible Graphene Oxide-Based Glucose Biosensors Langmuir, 2010, 26, 6158.
- Matyba, P.; Yamaguchi, H.; Eda, G.; Chhowalla, M.; Edman, L.; Robinson, N. D. Graphene and Mobile Ions: The Key to All-Plastic, Solution-Processed Light-Emitting Devices ACS Nano 2010, 4, 637.
- Becerril, H. A.; Mao, J.; Liu, Z.; Stoltenberg, R. M.; Bao, Z.; Chen, Y. Evaluation of Solution-Processed Reduced Graphene Oxide Films as Transparent Conductors ACS Nano 2008, 2, 463.
- Saha, S. K.; Bhaumik, S.; Maji, T.; Mandal, T. K.; Pal, A. J. Solution-Processed Reduced Graphene Oxide in Light-Emitting Diodes and Photovoltaic Devices with the Same Pair of Active Materials RSC Adv. 2014, 4, 35493.
- Li, S-S.; Tu, K-H.; Lin, C-C.; Chen, C-W.; Chhowalla, M. Solution-Processable Graphene Oxide as an Efficient Hole Transport Layer in Polymer Solar Cells ACS Nano 2010, 4, 3169.
- Wang, H.; Cui, L-F.; Yang, Y.; Casalongue, H. S.; Robinson, J. T.; Liang, Y.; Cui, Y.; Dai, H. Mn3O4-Graphene Hybrid as a High-Capacity Anode Material for Lithium Ion Batteries J. Am. Chem. Soc. 2010, 132, 13978.
- Yang, S.; Feng, X.; Ivanovici, S.; Müllen, K. Fabrication of Graphene-Encapsulated Oxide Nanoparticles: Towards High-Performance Anode Materials for Lithium Storage Angew. Chem. Int. Ed. 2010, 49, 8408.
- Lee, J. K.; Smith, K. B.; Hayner, C. M.; Kung, H. H. Silicon Nanoparticles–Graphene Paper Composites for Li Ion Battery Anodes Chem. Commun. 2010, 46, 2025.
- Zhou, G.; Wang, D-W.; Li, F.; Zhang, L.; Li, N.; Wu, Z-S.; Wen, L.; Lu, G. Q (M).; Cheng, H-M. Graphene-Wrapped Fe3O4 Anode Material with Improved Reversible Capacity and Cyclic Stability for Lithium Ion Batteries Chem. Mater. 2010, 22, 5306.
- Zhang, M.; Lei, D.; Yin, X.; Chen, L.; Li, Q.; Wang, Y.; Wang, T. Magnetite/Graphene Composites: Microwave Irradiation Synthesis and Enhanced Cycling and Rate Performances for Lithium Ion Batteries J. Mater. Chem. 2010, 20, 5538.
- Zhu, Y.; Murali, S.; Stoller, M. D.; Ganesh, K.; J.; Cai, W.; Ferreira, P. J.; Pirkle, A.; Wallace, R. M.; Cychosz, K. A.; Thommes, M.; Su, D.; Stach, E. A.; Ruoff, R. S. Carbon-Based Supercapacitors Produced by Activation of Graphene Science 2011, 332, 1537.
- Zhu, Y.; Murali, S.; Stoller, M. D.; Velamakanni, A.; Piner, R. D.; Ruoff, R. S. Microwave Assisted Exfoliation and Reduction of Graphite Oxide for Ultracapacitors Carbon 2010, 48, 2118.
- Liu, Z.; Robinson, J. T.; Sun, X.; Dai, H. PEGylated Nanographene Oxide for Delivery of Water-Insoluble Cancer Drugs J. Am. Chem. Soc. 2008, 130, 10876.
- Jung, H. S.; Kong, W. H.; Sung, D. K.; Lee, M-Y.; Beack, S. E.; Keum, D. H.; Kim, K. S.; Yun, S. H.; Hahn, S. K. Nanographene OxideHyaluronic Acid Conjugate for Photothermal Ablation Therapy of Skin Cancer ACS Nano. 2014, 8, 260.
- Yang, X.; Zhang, X.; Ma, Y.; Yi, H.; Wang, Y.; Chen, Y. Superparamagnetic graphene oxide–Fe3O4 nanoparticles hybrid for controlled targeted drug carriers J. Mater. Chem. 2009, 19, 2710.
- Lu, C-H.; Yang, H-H.; Zhu, C-L.; Chen, Z.; Chen, G-N. A Graphene Platform for Sensing Biomolecules Angew. Chem. Int. Ed. 2009, 48, 4785.
- Wang, Y.; Li, Z.; Hu, D.; Lin, C-T.; Li, J.; Lin, Y. Aptamer/Graphene Oxide Nanocomplex for in Situ Molecular Probing in Living Cells J. Am. Chem. Soc. 2010, 132, 9274.
- Song, Y.; Chen, Y.; Feng, L.; Ren, J.; Qu, X. Selective and Quantitative Cancer Cell Detection Using Target-Directed Functionalized Graphene and its Synergetic Peroxidase-like Activity Chem. Commun. 2011, 47, 4436.
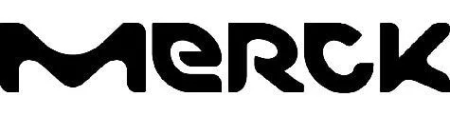
This information has been sourced, reviewed and adapted from materials provided by Merck.
For more information on this source, please visit Merck.