Glyphosate (N-(phosphonomethyl) glycine) is an organophosphorus compound that is used to kill weeds (e.g. annual broadleaf weeds and grasses) competing with crops.
Since first being introduced to market around 40 years ago, glyphosate is now one of the most widely used herbicides in the world due to its relatively low toxicity towards mammals compared to other herbicides.
After the introduction of genetically engineered “glyphosate tolerant”, the acceptance of glyphosate by farmers was further embraced. Unlike the weeds the herbicide is intended to destroy, crops such as corn and soybeans can withstand glyphosate treatment.
Like other pesticides, glyphosate is administered directly to food products, meaning both food workers and the environment may come into contact with the herbicide, resulting in the bio burden of exposure in unrestrained regional populations.
As a number of regulatory organizations have registered this herbicide product, glyphosate has been considered non-toxic with minimal risk to human health with sustained exposure at trace levels. However, recent assessments relative to toxicity by various organizations have placed glyphosate at the center of a controversy.
The World Health Organization's (WHO) International Agency for Research on Cancer classified it as “probably carcinogenic to humans” in March of 2015.1 However, in November 2015, the European Food Safety Authority (EFSA) released a report stating that there was no scientific evidence connecting glyphosate to cancer.2
Regardless of the dispute in the scientific community, federal regulations have been created by food authorities in several countries. The maximum residual level for glyphosate is typically somewhere between 0.05 to 500 mg/kg, but may differ depending on the food commodity.
Glyphosate is an extremely polar compound with high solubility in water and low solubility in the majority of organic solvents.
These properties mean that these compounds are not well retained on traditional C18 LC columns and non-polar GC columns.
As a result of this, the derivatization with fluorenylmethyloxycarbonyl chloride (FMOC-Cl) is a procedure commonly used to boost extraction and separation methods of glyphosate and other relative compounds with LC- and GC-based methods.
These derivatization-based methods are often labor-intensive, time-consuming and not as reproducible. There is a growing demand to develop a method to analyze levels of glyphosate and other polar compounds without the need for derivatization.
The EU Reference Laboratories (EURL) recently published two methods that can instantly analyze glyphosate (GLY), its metabolite, aminomethylphosphonic acid (AMPA), and glufosinate (GLU) without the need for derivatization.
One method utilized an ion exchange column with a prolonged run time of 23 minutes, while the second method used a Hypercarb column, which necessitates a specific priming/reconditioning procedure and demonstrated considerable amounts of chromatographic peak tailing.3
This study details a 12-minute LC/MS/MS method with an amino-based column to evaluate glyphosate and other related polar compound analysis in underivatized states, with superb selectivity and sensitivity.
Experimental
A PerkinElmer Altus® A-30 UPLC® system was used in combination with a PerkinElmer QSight™ 210 triple quadrupole mass spectrometer. Instrument control, data acquisition and processing was conducted utilizing the PerkinElmer Simplicity 3Q™ software. The LC method conditions are detailed in Table 1.
Table 1. LC method. Source: PerkinElmer Food Safety and Quality
. |
. |
Column |
Shodex NH2P-50 2D column, 2.0 x 150 mm, 5 μm |
Mobile Phase |
A: 5 mM ammonium acetate (pH11.0) in water;
B: acetonitrile |
Flow Rate |
0.25 mL/min. |
Oven Temp. |
35 ºC |
Injection Volume |
10 μL |
Gradient Conditions |
Time (min) |
Mobile Phase |
A (%) |
B (%) |
0.00 |
20 |
80 |
2.00 |
20 |
80 |
2.01 |
80 |
20 |
8.00 |
80 |
20 |
8.01 |
20 |
80 |
12.00 |
20 |
80 |
|
The mass spectrometer was fitted with an electrospray ionization source functioning in negative ion mode. The mass spectrometer source conditions are illustrated in Table 2:
Table 2. Mass spectrometer source conditions. Source: PerkinElmer Food Safety and Quality
Parameter |
Setting |
Dry Gas |
150 |
Nebulizer Gas |
220 |
Heating Gas Temp |
500 °C |
Electrospray Voltage |
-4500 V |
By infusing neat standard solutions, MRM settings for each analyte were optimized. Table 3 lists the parameters for each analyte’s MRM transition. The dwell time for each MRM was fixed at 30 ms.
Table 3. Optimized MRM settings. Source: PerkinElmer Food Safety and Quality
Compound |
Transitions m/z |
EV/V |
CE/eV |
(GLY) |
167.6/62.9* |
-19 |
35 |
167.6/149.6 |
14 |
(AMPA) |
109.7/63.0* |
-27 |
-30 |
109.7/78.9 |
37 |
(GLU) |
179.6/63.0* |
-20 |
53 |
179.6/84.9 |
27 |
* Quantifier ion
Sample Preparation
A 1 g oatmeal sample was weighed into a centrifuge tube; 10 mL of water/acetonitrile (V/V, 2/1) was added to the tube and the mixture was then shaken/vortexed for one minute, ultra-sonicated for 15 minutes, and centrifuged at 6000 rpm for five minutes.
The supernatant recovered was passed through a 0.22 μm nylon membrane filter for LC/MS/MS analysis. To prevent any potential interaction between analytes and glass surfaces, plastic sample vials were employed throughout analysis and samples were immediately analyzed after preparation.
Standards Calibration Solutions
Preparation of matrix-matched calibration standards was performed by adding varying levels of analytes (5.0, 10.0, 100.0, 200.0 and 500.0 ng/mL, respectively) in oatmeal matrix extract.
Results and Discussion
Figure 1 exhibits conventional MRM chromatograms for the three analytes spiked to 10 ng/mL (0.1 mg/kg) in oatmeal extract. All three analytes exhibited good peak shape and signal to noise as well as being well retained on the column. GLY and AMPA were eluted at almost identical retention times as a result of their similar chemical structure.
GLU was baseline divided from the other analytes. No matrix interferences were observed; these can impact peak integration.
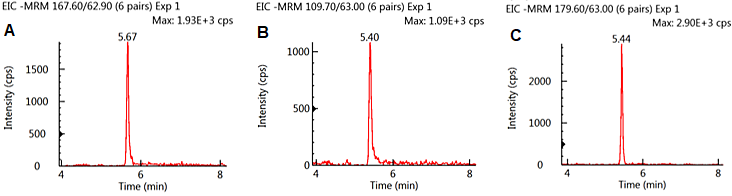
Figure 1. MRM Chromatograms of GLY (A), AMPA (B), and GLU (C) spiked at 10 ng/ml in oatmeal extract. Image Credit: PerkinElmer Food Safety and Quality
It is generally known that LC/MS/MS, particularly when working in ESI mode, is vulnerable to matrix effects, influencing the accuracy of quantitation.
Throughout this study, a comparison of signal intensities of standards in neat solution with those of standards in matrix-matched solution at varying concentration levels was conducted to calculate matrix effects (ME).
Table 4. Matrix effect result in oatmeal matrix. Source: PerkinElmer Food Safety and Quality
Compound |
GLY |
AMPA |
GLU |
Matrix effect (%) |
67.3 |
87.3 |
107.6 |
An ME value less than 100% signifies matrix suppression, whereas an ME value greater than 100% implies matrix enhancement. As shown in Table 4, both GLY and AMPA demonstrate matrix suppression, while GLU exhibits matrix enhancement. Utilizing matrix-matched standards, it is possible to compensate for matrix effects, which may facilitate good quantitational accuracy without using internal standards.
Calibration curves were produced by running matrix-matched calibration standards as detailed in the experimental section.
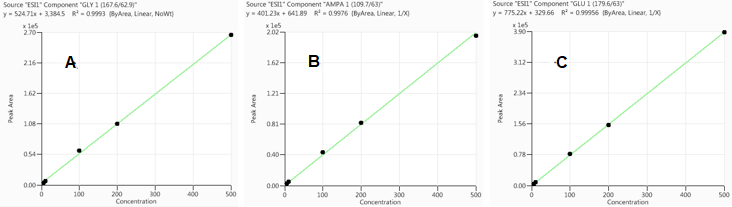
Figure 2. Calibration curves for GLY (A), AMPA (B) and GLU (C) in oatmeal extract, respectively. Image Credit: PerkinElmer Food Safety and Quality
Figure 2 displays the calibration curves for GLY, AMPA and GLU. Respectable linear correlation coefficients (R2 ≥0.997) were acquired between concentrations of 5 to 500 ng/mL (0.05-5 mg/kg in real sample).
For the 5 ng/mL calibrant, the signal-to-noise ratios (S/N) for GLY, AMPA, and GLU were 432, 165, and 325, respectively. Using these values, the limits of quantitation (LOQs; S/N ≥ 10) were determined to be 0.12, 0.30 and 0.15 ng/mL. As the EU has declared that the maximum residue limit (MRL) for glyphosate in oatmeal is 20 mg/kg, the method derived from this study clearly meets this standard.
Table 5. Linear dynamic range, regression coefficients, LOQ and S/N at LOQ level for analytes. Source: PerkinElmer Food Safety and Quality
Compound |
Range
(ng/mL) |
R2 |
S/N at
5 ng/mL |
LOQ in matrix
(S/N ≥ 10) Ng/mL |
GLY |
5-500 |
0.999 |
432 |
0.12 |
AMPA |
5-500 |
0.997 |
165 |
0.30 |
GLU |
5-500 |
0.999 |
325 |
0.15 |
Table 6. Recovery of the analytes from oatmeal sample at different concentration levels. Source: PerkinElmer Food Safety and Quality
Compound |
Spiked Level (50 μg/kg) |
Spiked Level (1 mg/kg) |
Recovery /% |
Recovery /% |
Recovery /% |
RSD/% |
GLY |
118 |
8.74 |
85 |
6.91 |
AMPA |
130 |
11.8 |
94 |
8.72 |
GLU |
122 |
12.9 |
96 |
1.93 |
Conclusion
This study announces a rapid, sensitive, and reliable 12-minute LC/MS/MS method that facilitates direct analysis of GLY, AMPA, and GLU in oatmeal without derivatization.
The sample preparation method was a straightforward water/acetonitrile extraction, which demonstrated good recoveries and the minimum amount of matrix effects for all three compounds.
The calibration curves for three analytes demonstrated excellent linearity over three orders of magnitude with a calibration fit of R2 greater than 0.997.
The LOQs for glyphosate and other relative polar compounds were significantly less than the EU’s MRL of 20 mg/kg in oatmeal.
References
- http://monographs.iarc.fr/ENG/Monographs/vol112/ mono112-09.pdf. Accessed on Aug 2nd, 2016
- Conclusion on the peer review of the pesticide risk assessment of the active substance glyphosate. EFSA Journal 2015; 13(11):4302.
- Quick Method for the Analysis of numerous Highly Polar Pesticides in Foods of Plant Origin via LC-MS/MS involving Simultaneous Extraction with Methanol (QuPPe-Method). http://www.crl-pesticides.eu/userfiles/ file/EurlSRM/meth_QuPPe-PO_EurlSRM.pdf. Accessed on Aug 2nd, 2016
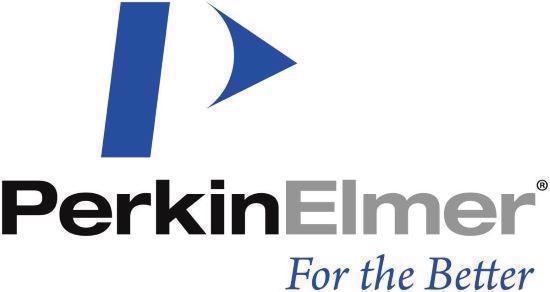
This information has been sourced, reviewed and adapted from materials provided by PerkinElmer Food Safety and Quality.
For more information on this source, please visit PerkinElmer Food Safety and Quality.