This study is focused on filament-based microrheology (FMR), employing high-aspect-ratio semi-flexible strands as probes.
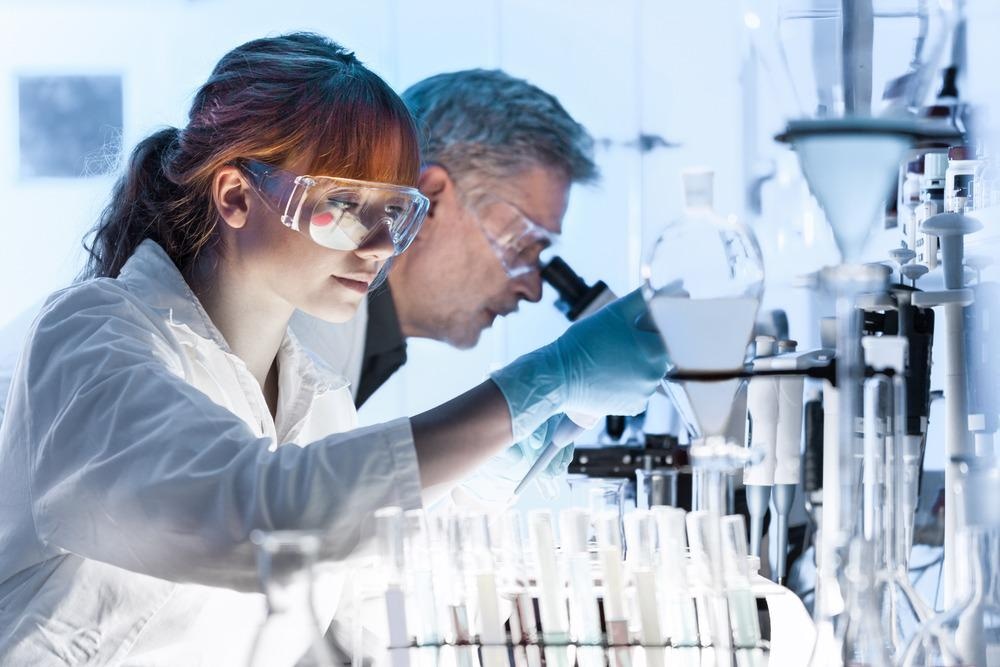
Image Credit: Matej Kastelic/Shutterstock.com
Microrheology is a new technology that employs minuscule tracers to investigate structural parameters on a short size range. Microrheology may investigate the mechanical and physical characteristics of natural fibers on microscopic length scales. A popular method involves tracking the oscillations of micrometer-sized pellets inserted in the substance to be described.
When the substrate retains complexity on equivalent size scales, this method produces findings that rely on probe length. The novel probes in this research are far less intrusive than conventional beads because of their narrow cross-sections.
Significant Types of Microrheology
Microrheology is classified into two distinct kinds: passive microrheology and active microrheology. Passive microrheology moves fluorescent probes using intrinsic heat energy, whereas active microrheology employs the application of external forces and fields, such as a magnetic flux or ocular pliers.
Microrheology is further subdivided into 1- and 2-particle approaches. Rheological characteristics are acquired in active microrheology by forcing microscopic probing nanoparticles in the medium to move, which can extend investigations from the linear to the non-linear domain.
Linear viscoelastic characteristics are acquired in passive microrheology from the mobility of microscopic probing nanoparticles enduring temperature fluctuations in a medium at a thermal equilibrium state; that is, no extrinsic forces are applied to the probing nanoparticles.
Industrial Utilization in the Past
Microrheology is a technology for probing the nanoscale elastomeric characteristics and kinematics of soft materials by watching the mobility of nanoparticles placed inside them. Soft materials, such as surfactant- or polymeric mixtures, generally contain morphology exceeding the atomic size and have distinct relaxation durations ranging from milliseconds to hours or more.
The quantitative research method of the mechanical characteristics of living cells is an important application of microrheology. This method is known as bio-microrheology (BMR), and it is dependent on the use of microrheology approaches that were created for the investigation of homogenous complicated liquids.
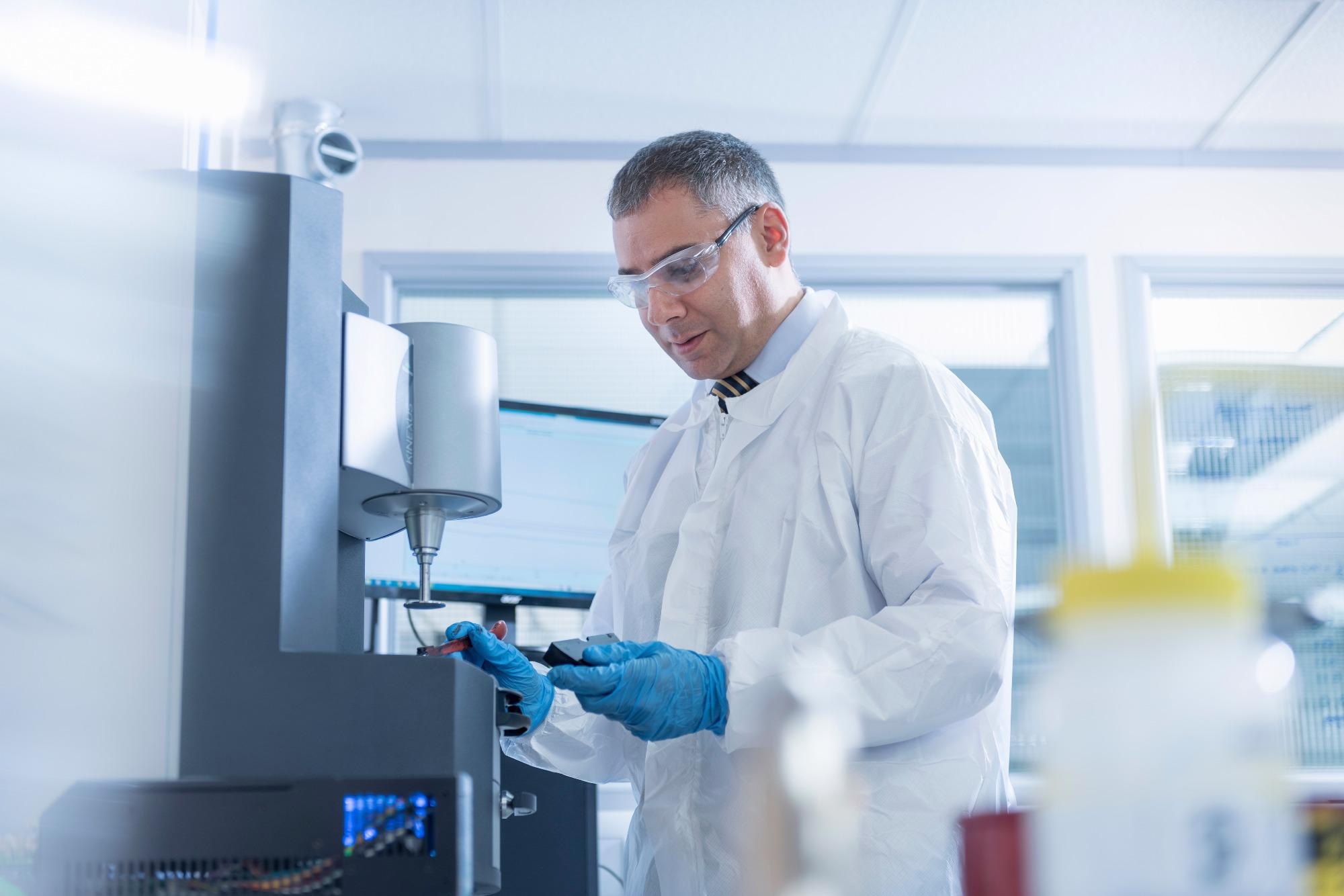
Image Credit: Image Source Trading Ltd/Shutterstock.com
Microrheology may also be used in microscopic particles mobility studies, in which complex fluids are subjected to regulated circulation and displacement operations in sub-millimeter scale circuits to acquire viscoelastic parameters.
Limitations of Microrheology
Certain limitations and disadvantages of microrheology have been identified which are key points to consider while considering its industrial utilization. The frequency range of operation is very limited, whilst the moduli that can be probed are also very limited. Another significant disadvantage is the capability to address geographical heterogeneity. It is challenging to investigate the nonlinear behavior using microrheology. Furthermore, microrheology can only be used for materials that are at least partly translucent to light.
The current work introduces a unique usage of thin filaments, or moderately flexible polymers, as localized stealth probes. Filaments incorporated in an elastomeric structure, such as microtubules in the cellular cytoskeleton, pulsate in response to the network's temperature or kinetic movements.
Research Findings
The latest study in Physics Revision Letters considers FMR. Single-walled carbon nanotubes (SWNTs) with exceptional dimensions, a diameter of 1 nm, and lengths of tens of µm, are used for the research. Decomposition of recorded changing SWNT forms into dynamic twisting eigenvalues was performed. Each phase is responsive to material qualities on the wavelength range.
The researchers used the five-point stencil approach to generate the arithmetic differential, together with Simpson's formula for the following integral, to boost performance. Modal peaks varied by about 0, as predicted for an innately linear filament.
The magnitude of the applied loads declined, as anticipated from equipartition for thermally induced phases. For the very first three forms, the twisting oscillations of SWNTs were much bigger than the background noise level. It was observed that all MSADs display a simple linear gradient 1 for short intervals up to 1 s, demonstrating the system's viscoelastic behavior.
Mixtures with solvent intensities ranging from 1 to 4.5 mg/ml were comprehensively investigated to validate Filament Microrheology (FMR) in a viscous fluid. The filaments' thermal changes were observable, and complicated shear elastic moduli were determined.
Interfacial shear moduli estimated from many phases accord as anticipated at each intensity. Flexural and shear elastic modulus rose as HA concentration was increased. Hence, all results were in accordance with theoretical concepts confirming the advantages and functional efficiency of filament-based microrheology.
Future Perspective
Interfacial shear elastic modulus estimated from many phases accord as anticipated at each intensity. Flexural and shear elastic modulus rose as HA concentration was increased. This confirms its viability for various industrial utilizations in the near future such as diffusion spectroscopy, actin filament networks, high-frequency analysis, etc.
In short, FMR is exceptionally valuable for determining the magnitude-dependent viscoelasticity of soft materials with organizational frameworks, such as the cytoskeleton of live cells. The findings showed excellent compatibility with traditional microrheology spanning nearly two magnitudes in periodicity.
Other moderately flexible filaments, such as actin filaments or microtubules, are predicted to be employed as sensors in biological systems, making them fully non-invasive and avoiding the admission of foreign items into cells. The method also indicates alternative modifications that may be used to evaluate the rheological characteristics of the external media by exploiting the shape changes of other structures such as membranes.
References
Nishi, K., MacKintosh, F. C., & Schmidt, C. F. (2021). Multi-scale microrheology using fluctuating filaments as stealth probes. Physical Review Letters. https://journals.aps.org/prl/abstract/10.1103/PhysRevLett.127.158001
Nishizawa, K., Honda, N., Ikenaga, M., Inokuchi, S., Sugino, Y., Arigac, T., & Mizuno, D. (2021). Feedback Microrheology in Soft Matter. Soft Condensed Matter. https://arxiv.org/abs/2106.05119
Rizzi, L. G. (2020). Microrheological approach for the viscoelastic response of gels. Journal of Rheology. https://sor.scitation.org/doi/10.1122/8.0000034
Waigh, T. A. (2016). Advances in the microrheology of complex fluids. Reports on Progress in Physics. https://iopscience.iop.org/article/10.1088/0034-4885/79/7/074601
Disclaimer: The views expressed here are those of the author expressed in their private capacity and do not necessarily represent the views of AZoM.com Limited T/A AZoNetwork the owner and operator of this website. This disclaimer forms part of the Terms and conditions of use of this website.