AZoM speaks to Michael Jewett, a researcher at Northwestern University, about a novel process using bacteria to capture CO2 and convert it into the useful commercial chemicals acetone and isopropanol. This could bring us closer to a circular bioeconomy in the chemical sector.
Please can you introduce yourself and how you came to research biotechnology?
My name is Michael Jewett. I am a professor in chemical and biological engineering at Northwestern University, where I also direct the Center for Synthetic Biology. I always loved chemistry, biology, and math, which led me to chemical engineering as a discipline.
While a Ph.D. student at Stanford University, I started research in biotechnology, having worked with Jim Swartz who was at Genentech before starting his academic career. Since then, I have sought ways to fundamentally advance and apply our technological capacities to partner with biology to make what is needed, where and when it is needed, and on a sustainable and renewable basis.
With an increasing amount of research focused on tackling the climate crisis, how significant is the control of carbon emissions to these efforts?
We have a climate emergency, all linked to the unabated release and accumulation of CO2 in the atmosphere.
Solutions for carbon-free, renewable production of energy exist, but most of the things around us and everyday products are based on hydrocarbon chemicals produced from fresh fossil sources.
Thus, we need to develop new carbon-negative strategies to make chemicals and products. This is significant to fighting the climate crisis, and microbes can tackle some of the toughest-to-decarbonize sectors.
In your latest research, an optimized bacteria strain was engineered that can convert Co2 into acetone and isopropanol. Could you please outline the process through which this is possible?
Our process is based on a special microorganism (Clostridium autoethanogenum) that is able to feed on carbon oxide gases. The process is similar to the fermentation of beer and wine, but instead of sugars and yeast, we use waste gases and this microorganism.
The microorganism C. autoethanogenum is naturally capable of producing ethanol from these waste gases, and LanzaTech is already successfully operating two commercial plants converting emissions from the heavy industry into ethanol. To date over 30M gallons of ethanol have been produced and over 150,000 tons of CO2 avoided.
We achieved our goals using synthetic biology, which allows researchers to reprogram organisms for social good (e.g. yeast has been engineered to produce the anti-malaria drug artemisinin). While genetic engineering is now carried out routinely in yeast or E. coli, the microorganism we work with was considered genetically inaccessible just a decade ago. Thus, the advent of new tools was critical to our work being successful.
What inspired you to consider using bacteria as carbon converters?
We considered bacteria because some are already natural carbon converters. In this case, we just had to reprogram the bacteria to make a new sustainable product. We used the processes of biology (i.e., carbon fixation) to make products this bacteria had never made before.
How were you able to specifically engineer bacteria to produce these results?
To succeed in engineering the organism from making ethanol to making acetone and isopropanol, we developed a new framework for biosystems design.
In a first step, we mined for enzymes that allow the production of acetone or isopropanol from a common intermediate in metabolism (acetyl-CoA). We then introduced these genes and tested different variants to optimize production.
When we first introduced the acetone pathway we only saw very little acetone production, but surprisingly other products such as 3-hydroxybutyrate appeared. We traced this back to interactions of the introduced acetone pathway with the native metabolism. It can be very time-consuming to identify which parts of the native metabolism are responsible for this.
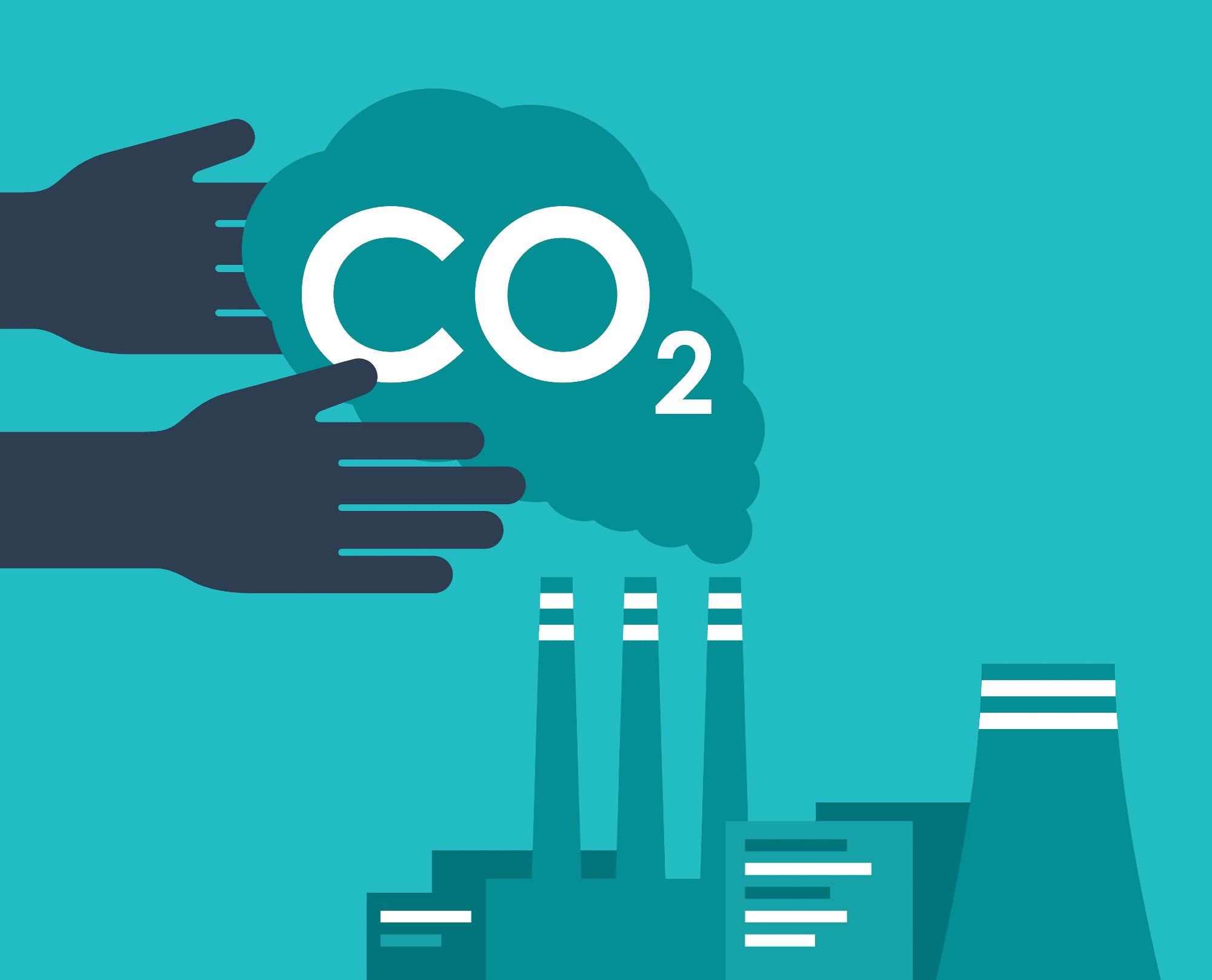
Image Credit: Dmitry Kovalchuk/Shutterstock.com
Using computational analysis we could narrow this down to a set of 15 enzymes, but it would have still taken months to years to test any of these combinations. Thus, we used cell-free tools (the ability to boot up biochemical reactions without living organisms) to accelerate our design process. Cell-free tools make it so that processes that would have normally taken 6-12 months took just weeks.
Finally, we developed a process that can operate stably and at high rates for weeks at a time, and scaled this process from the lab bench to an in-field industrial pilot that resembles LanzaTech’s commercial plant.
How widely are acetone and isopropanol used, and how significant will their clean production be to a circular bioeconomy?
Acetone and isopropanol are important commodity chemicals with established markets of > $10 billion. For example, both molecules are industrial solvents as well as platform chemicals for the production of materials such as acrylic glass (polymethyl methacrylate) and polypropylene.
A key feature of these molecules is that they can be separated using similar technologies to ethanol, which allows us to use the same plant infrastructure at LanzaTech and switch between products (e.g., from ethanol to acetone or isopropanol) by simply changing the microbe. This is a paradigm shift for the chemical industry, where a plant is typically purpose-built for a certain product and production cannot be easily changed. We believe this approach is significant towards a circular economy.
How significant do you believe this two-fold contribution of Co2 reduction and clean chemical production will be to reducing global carbon emissions?
It is very significant. The engineered bacteria ferment carbon dioxide from the air to produce the chemicals acetone and isopropanol. Manufacturing these chemicals using conventional processes results in emissions of ~2 kg CO2/kg product along with the accumulation of other toxic waste and, before now, no sustainable, green chemistry alternative existed. We have developed a process that enables carbon-negative manufacturing of these chemicals, effectively pulling CO2 out of the atmosphere and locking ~1.5 kg of CO2 into the product per kg produced.
Are there any limitations that are yet to be overcome in the fermentation process?
We need to demonstrate the process beyond a pilot scale. In addition, we are curious to see if our approach can be readily adapted to a wide range of commodity chemicals.
What is personally the most exciting or significant aspect of this research to you?
What’s so exciting is that we believe that the framework developed here will provide a blueprint for the development of further carbon-negative chemical production processes.
More broadly, our work highlights how synthetic biology has the potential to be a central pillar of a global strategy for enabling people and the planet to flourish in partnership.
What are the next steps for your research team? Will you continue to research clean biotechnologies?
We are in the process of scaling up this technology. Our vision for commercialization is to transform established ethanol-producing gas fermentation facilities into product-flexible production plants. By swapping the ethanol-producing microbe currently deployed in our commercial gas fermentation facilities, with a new microbe programmed for acetone or propanol production, we can instantly increase the range of products that an individual facility can make.
This product flexibility will enable plant operators to make market-based decisions on which products to focus on at any time.
Where can readers find more information?
Proof of concept of a wide range of molecules that have been demonstrated already in these organisms, e.g. https://doi.org/10.1016/j.copbio.2020.02.017
Manuscript - https://www.nature.com/articles/s41587-021-01195-w
LanzaTech - https://www.lanzatech.com/
Jewett Lab - http://jewettlab.northwestern.edu/
Stories:
https://www.nature.com/articles/d41586-022-00488-7
https://www.science.org/content/article/microbes-convert-industrial-waste-gases-commodity-chemicals
https://www.newscientist.com/article/2309078-engineered-bacteria-produce-chemicals-with-negative-carbon-emissions/
About Michael Jewett
Michael Jewett is the Charles Deering McCormick Professor of Teaching Excellence, the Walter P. Murphy Professor of Chemical and Biological Engineering, and the Director of the Center for Synthetic Biology at Northwestern University. Dr. Jewett received his Ph.D. in 2005 at Stanford University, completed postdoctoral studies at the Center for Microbial Biotechnology in Denmark and the Harvard Medical School, and was a guest professor at the Swiss Federal Institute of Technology (ETH Zurich). He is the recipient of the NIH Pathway to Independence Award, David and Lucile Packard Fellowship in Science and Engineering, Camille-Dreyfus Teacher-Scholar Award, and a Finalist for the Blavatnik National Awards for Young Scientists, among others. He is the co-founder of SwiftScale Biologics, Stemloop, Inc., Pearl Bio, and Design Pharmaceuticals. Jewett is a Fellow of AIMBE, AAAS, and NAI.
Disclaimer: The views expressed here are those of the interviewee and do not necessarily represent the views of AZoM.com Limited (T/A) AZoNetwork, the owner and operator of this website. This disclaimer forms part of the Terms and Conditions of use of this website.