The food industry assesses the nutritional content of milk-based powders, such as infant formula, which are made with additional nutrients, using many analytical procedures. Proteins, lipids, carbs, vitamins, and minerals are a few nutrients in these “fortified” milk powders.
The elemental composition of milk-based powders is regulated near the production line using energy-dispersive X-Ray fluorescence (EDXRF).
To streamline manufacturing procedures and reduce the turnaround time, quick sample preparation and measurement times are essential. This article monitors the following elements using EDXRF: Na, Mg, P, Cl, K, Ca, Mn, Fe, Cu, and Zn.
Due to their lower sensitivity, Na and Mg remain challenging for EDXRF. Here, it is shown how utilizing a silicon drift detector (SDD) with a graphene window could significantly increase their detection efficiency.
Instrumentation
The end-window X-Ray tube of the Thermo Scientific™ ARL QUANT’X™ EDXRF Spectrometer is calibrated at 50 Watts, and the target can be either Rh or Ag. With primary beam filters, the sample was examined using a direct excitation geometry. There are a total of nine filters that can be used to enhance element excitation.

Image credit: Thermo Fisher Scientific - Elemental Analyzers and Phase Analyzers
All periodic table elements, from carbon (Z = 6) to Am (Z = 95), can be detected using a large-area SDD with a graphene window (less than 1 µm thick). Until recently, the standard SDD window was an 8 µm thick Be window, which prevented the detection of elements lighter than F (Z = 9).
Sample Preparation
Using a die set constructed of hardened stainless steel, 6.0 ± 0.1 g of powder in each sample was crushed into a pellet with a 32 mm diameter. Around four tons of pressure was applied using a manual hydraulic press made by Specac in the United Kingdom. Before pelletizing, samples were not ground or dried. A desiccator was used to store the pellets.
The resultant pellets were >5 mm thick. With regard to the depth of the distinctive X-Rays of interest for each element, this could be thought of as infinitely thick. Pellets were, therefore, examined from both sides and considered true duplicates.
There were 36 internal milk-based powder standards created for this application. The validation phase required the use of the remaining 23 standards, whereas the calibration phase required the use of 13 standards.
Measurement Conditions
The measurement of 10 elements was carried out under three excitation settings. The experimental data for each condition are shown in Table 1.
Table 1. Excitation conditions used in this application note. Source: Thermo Fisher Scientific-Elemental Analyzers and Phase Analyzers
Condition |
Voltage
(kV) |
Current
(mA) |
Filter |
Atmosphere |
Live Time
(s) |
Analytes |
ROI Normalizing Background
(keV) |
Low Za |
4 |
2.00 (Auto) |
No Filter |
Helium |
180 |
Na, Mg |
|
Low Zb |
8 |
2.00 (Auto) |
C Thick |
Helium |
60 |
P, S, K, Ca |
4.4 - 6.0 |
Mid Zb |
20 |
2.00 (Auto) |
Ag Medium |
Air |
180 |
Mn, Fe, Cu, Zn |
13.8 - 17.1 |
Lighter elements are tested in helium to prevent air from absorbing their low-energy X-Rays’ distinctive properties. Due to the tendency of milk powder particles to enter the vacuum pump and impact the oil quality, analysis in helium is preferable over the vacuum.
With 180 seconds each devoted to the two conditions, which focused on light and transitional aspects, the total measurement period was 420 seconds of “live time.”
The measurement duration can be shortened depending on the level of uncertainty and reproducibility required, particularly in the case of the condition targeted at transition elements where Mn concentrations are close to the detection limit. The measurement period for such circumstances can be cut to 60 seconds if the determination of Mn is not important.
Calibration
To set up the calibration, a total of 13 in-house standards that have previously undergone inductively coupled plasma optical emission spectroscopy (ICP-OES) analysis are employed. Standards are measured twice (both sides of the pellet), and the concentration ranges for each element are shown in Table 2.
Table 2. Elemental concentration ranges and calibration statistics. Source: Thermo Fisher Scientific-Elemental Analyzers and Phase Analyzers
Element |
Conc. range
(ppm) |
RMS
(ppm) |
R2 |
Na |
1400 - 4920 |
157 |
0.979 |
Mg |
358 - 1212 |
56 |
0.961 |
P |
1670 - 5160 |
172 |
0.980 |
Cl |
2950 - 5830 |
126 |
0.989 |
K |
4290 - 11250 |
159 |
0.995 |
Ca |
2550 - 7570 |
231 |
0.985 |
Mn |
0.3 - 13 |
0.4 |
0.994 |
Fe |
51 - 97 |
3.0 |
0.968 |
Cu |
3 - 8 |
0.3 |
0.966 |
Zn |
34 - 95 |
1.9 |
0.989 |
To calculate net elemental peak intensities, spectral fitting is employed to account for background and spectral overlap.
In certain situations, the ratio of the peak intensity to a part of the dispersed spectral background was determined and utilized in place of only the element intensity to account for the matrix effect brought on by variations in the organic content of the powders and to enhance the calibration model.
Not all elements had this ratio applied since it is not always beneficial. This normalization was successful for the elements K, Ca, Mn, Fe, Cu, and Zn.
The calibration curves for the various elements are displayed in Figure 1. The calibration’s root mean square error (RMSE) and correlation coefficient (R2) for each element are also displayed in Table 2.
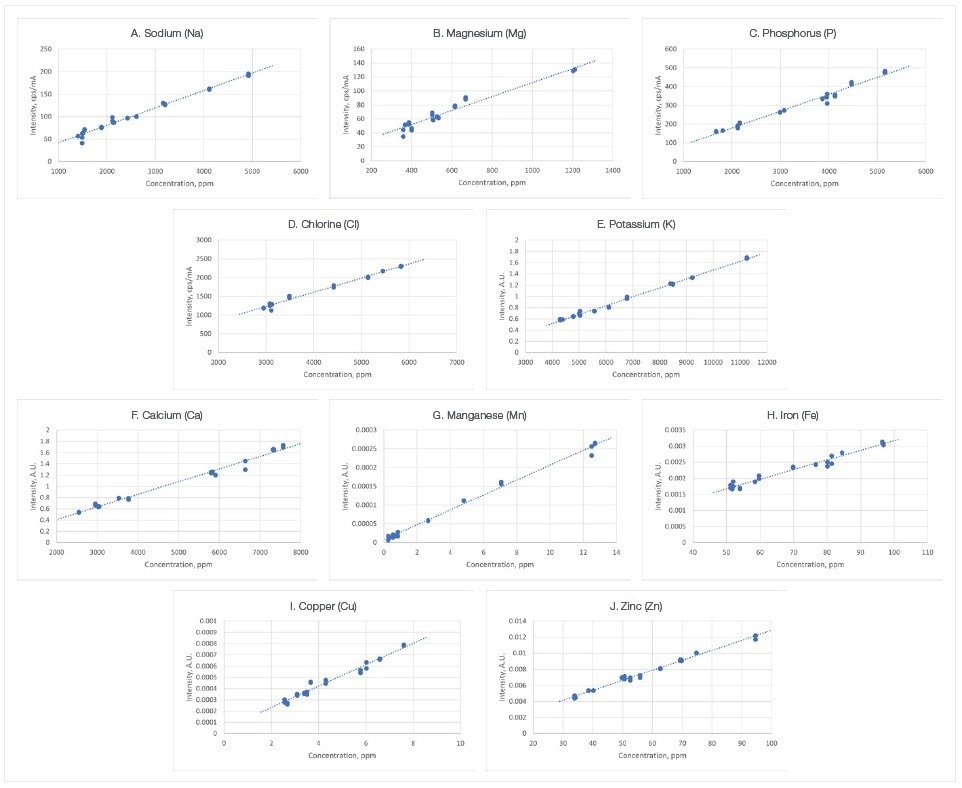
Figure 1. Elemental calibration curves as obtained with EDXRF. Image Credit: Thermo Fisher Scientific-Elemental Analyzers and Phase Analyzers
Figure 2 displays the spectrum for one of the standards that had an excitation condition of Low Za and included 1400 ppm Na and 500 ppm Mg (Table 1). The SDD with a graphene window produces element peaks with high statistics and has exceptional sensitivity for Na and Mg.
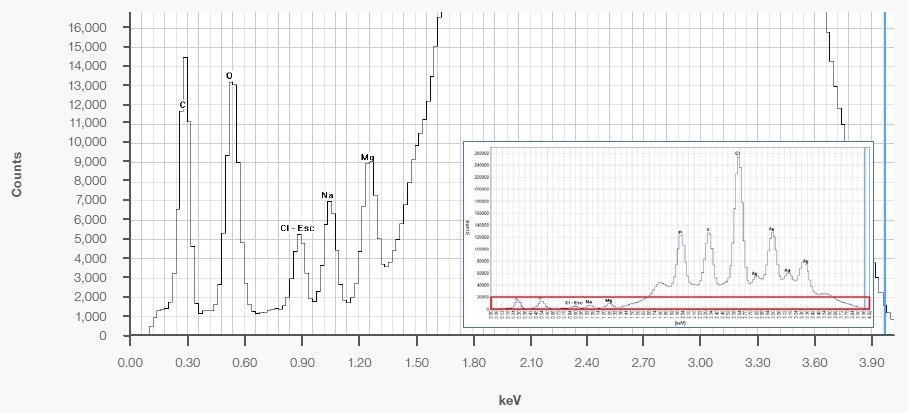
Figure 2. Spectrum of a milk-based powder containing 1,400 ppm Na and 500 ppm Mg, obtained using condition “Low Za” as shown in Table 1 (4 kV, no filter, 180 seconds live time). Image Credit: Thermo Fisher Scientific-Elemental Analyzers and Phase Analyzers
As a result, it is now feasible to create calibration curves with low RMS values, which was previously not achievable with EDXRF.
Validation
To verify the EDXRF technique, a total of 23 internal standards that had previously undergone ICP-OES analysis were employed. Each standard underwent two measurements. Figure 3 compares the ICP-OES results with the EDXRF data (the average of each duplicate).
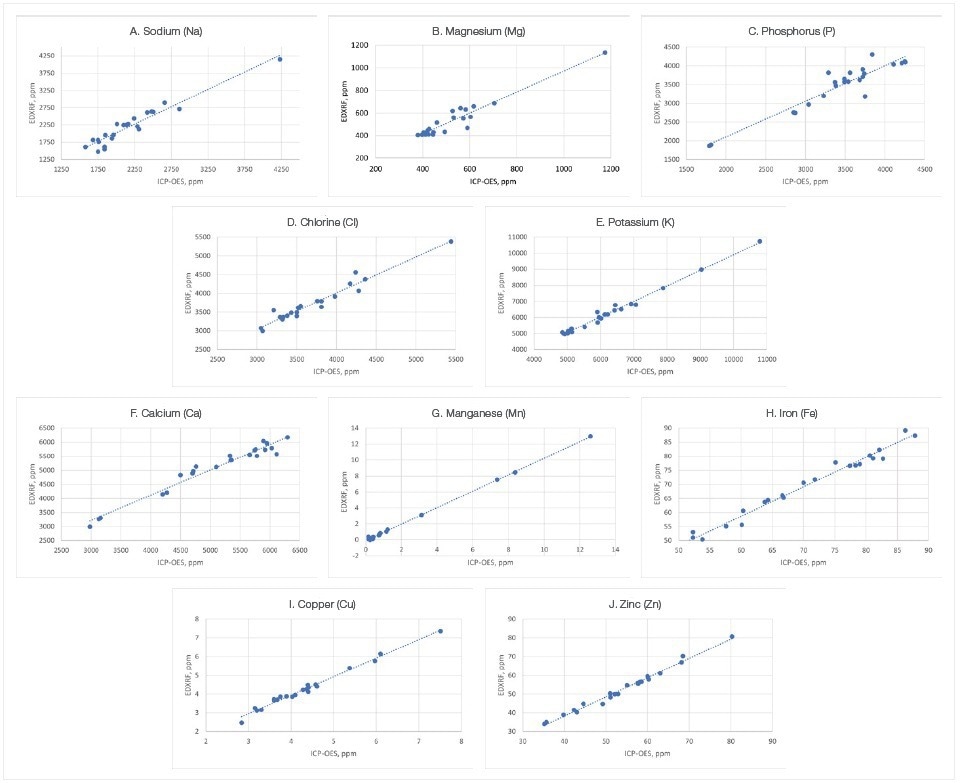
Figure 3. Milk-based powder standards analyzed with EDXRF, as compared to ICP-OES results. Image Credit: Thermo Fisher Scientific-Elemental Analyzers and Phase Analyzers
Standard error of prediction (SEP), correlation coefficient (R2), slope, intercept, standard deviations (SD) of the slope and intercept, and confidence intervals (CI) are only a few of the statistical metrics that are pertinent for all elements and are shown in Table 3.
Table 3. Validation statistics. Source: Thermo Fisher Scientific-Elemental Analyzers and Phase Analyzers
|
Na |
Mg |
P |
Cl |
K |
Ca |
Mn |
Fe |
Cu |
Zn |
SEP |
162 |
47 |
221 |
131 |
158 |
203 |
0.16 |
2.0 |
0.14 |
1.9 |
R2 |
0.921 |
0.920 |
0.886 |
0.945 |
0.988 |
0.960 |
0.999 |
0.980 |
0.985 |
0.987 |
Slope |
1.005 |
0.929 |
0.948 |
0.961 |
0.967 |
0.900 |
1.033 |
1.047 |
0.987 |
1.027 |
SD of slope |
0.064 |
0.060 |
0.074 |
0.053 |
0.024 |
0.040 |
0.008 |
0.032 |
0.027 |
0.026 |
CI Min |
0.872 |
0.805 |
0.794 |
0.849 |
0.918 |
0.817 |
1.016 |
0.980 |
0.932 |
0.973 |
CI Max |
1.139 |
1.054 |
1.102 |
1.073 |
1.017 |
0.984 |
1.050 |
1.115 |
1.042 |
1.081 |
Intercept |
13 |
42 |
213 |
169 |
227 |
516 |
-0.09 |
-4.1 |
-0.01 |
-2.8 |
SD of intercept |
145 |
33 |
259 |
200 |
152 |
207 |
0.03 |
2.3 |
0.12 |
1.4 |
CI Min |
-289 |
-26 |
-326 |
-250 |
-89 |
85 |
-0.15 |
-8.9 |
-0.25 |
-5.8 |
CI Max |
314 |
110 |
751 |
589 |
543 |
947 |
-0.03 |
0.6 |
0.24 |
0.1 |
When the fitted straight line has a slope of 1 and an intercept of 0, taking the confidence intervals for both parameters into account, a strong statistical agreement is attained. The majority of the items in Table 3 meet these requirements. Ca and Mn do not satisfy the requirements for the slope and intercept.
This is probably due to the concentration range in the case of Mn, which is near the technique’s detection limit. Since various material volumes were sampled for the EDXRF and ICP-OES measurements, it is assumed that Ca is not as uniformly distributed throughout the material as the other elements, which results in inconsistencies between the data.
Alternately, compensating for matrix effects might not be possible by normalizing to a portion of the spectral background. The slope and intercept values, however, practically satisfy the standards, and the agreement between the EDXRF and ICP-OES results is satisfactory.
Stability Over Several Days
To evaluate the stability of EDXRF over time, five validation samples were routinely examined over the course of 28 days (Table 4). Each concentration presented is an average of replicates.
Table 4. Analysis of a validation sample over a period of 28 days. Source: Thermo Fisher Scientific-Elemental Analyzers and Phase Analyzers
Element |
Ref.
ppm |
Day 1
ppm |
Day 7
ppm |
Day 9
ppm |
Day 12
ppm |
Day 14
ppm |
Day 16
ppm |
Day 19
ppm |
Day 21
ppm |
Day 23
ppm |
Day 26
ppm |
Day 28
ppm |
Na |
2160 |
2202 |
2156 |
2164 |
2143 |
2200 |
2196 |
2195 |
2190 |
2183 |
2188 |
2205 |
Mg |
417 |
429 |
414 |
413 |
417 |
421 |
418 |
428 |
418 |
425 |
421 |
417 |
P |
2850 |
2678 |
2715 |
2691 |
2698 |
2727 |
2703 |
2719 |
2688 |
2688 |
2678 |
2704 |
Cl |
3330 |
3277 |
3280 |
3263 |
3290 |
3304 |
3312 |
3326 |
3287 |
3306 |
3298 |
3290 |
K |
4920 |
4871 |
4860 |
4869 |
4863 |
4902 |
4898 |
4882 |
4877 |
4887 |
4884 |
4891 |
Ca |
4270 |
4160 |
4174 |
4175 |
4181 |
4182 |
4207 |
4193 |
4199 |
4210 |
4204 |
4206 |
Mn |
1.2 |
1.2 |
1.2 |
1.2 |
1.2 |
1.4 |
1.5 |
1.3 |
1.2 |
1.6 |
0.9 |
1.2 |
Fe |
52.3 |
54.6 |
54.2 |
53.9 |
54.0 |
54.5 |
54.1 |
54.0 |
54.1 |
54.1 |
53.8 |
54.0 |
Cu |
4.3 |
4.2 |
4.2 |
4.2 |
4.3 |
4.2 |
4.2 |
4.3 |
4.3 |
4.2 |
4.2 |
4.2 |
Zn |
52.1 |
49.4 |
48.7 |
49.2 |
49.0 |
49.2 |
48.6 |
49.3 |
48.8 |
49.2 |
49.1 |
49.1 |
Figure 4 displays the outcomes for a validation sample that was tracked for 28 days. Even for elements present at lower ppm concentrations, the QUANT’X EDXRF Spectrometer generates consistent analytical findings.
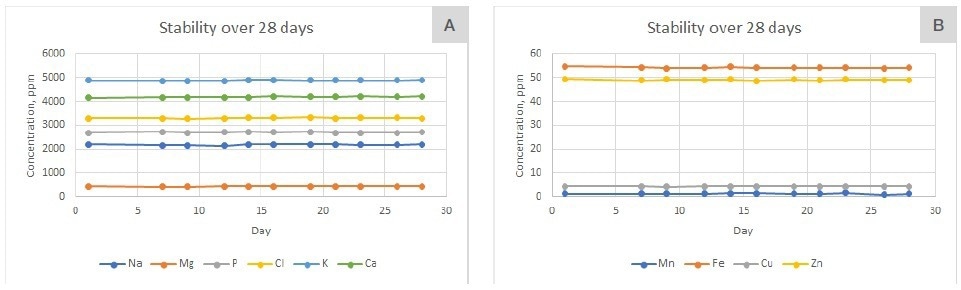
Figure 4. Analysis results over 28 days. A) Elements present at concentrations >500 ppm. B) Elements present at concentrations <100 ppm. Image Credit: Thermo Fisher Scientific-Elemental Analyzers and Phase Analyzers
Summary
When fitted with a graphene detector window, the ARL QUANT’X EDXRF Spectrometer becomes an excellent instrument for analyzing the elemental composition of milk-based powders. Previously difficult-to-detect light components, such as Na and Mg, now exhibit well-defined signals with enough intensity to produce reliable calibration curves.
This tech note demonstrates how in-house standards are utilized to build up a calibration, which is followed by a thorough validation. The elemental peak intensity is normalized by a portion of the spectral background to account for any matrix effects, eliminating inter-element adjustments, and creating simple and reliable calibration models.
The ARL QUANT’X Spectrometer’s performance during a 28-day period demonstrates high stability over time, which minimizes the need for drift correction or recalibration.
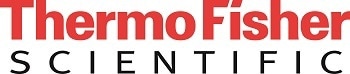
This information has been sourced, reviewed and adapted from materials provided by Thermo Fisher Scientific - Elemental Analyzers and Phase Analyzers.
For more information on this source, please visit Thermo Fisher Scientific - Elemental Analyzers and Phase Analyzers.