The glass transition of polymer materials is a crucial factor that affects material properties. Typically, this transition is analyzed using Differential Scanning Calorimetry (DSC).
However, sometimes the identification of the glass transition becomes complicated when it overlaps with endothermic processes, such as evaporation and enthalpy relaxation, occurring in the same temperature range.
To overcome this challenge, a technique known as Temperature Modulated DSC (TM-DSC) is used. This technique combines periodic temperature control performed by AC calorimetry with constant rate temperature control performed by DSC measurement.
By separating the effects of non-reversing phenomena such as evaporation and enthalpy relaxation, TM-DSC is an effective technique for clearly observing the glass transition.
What is DSC?
Differential scanning calorimetry (DSC) is a thermal analysis technique in which the difference between the rate of heat flow into a specimen and that into a reference is measured as a function of temperature or time while the specimen and reference are exposed to the same temperature control program.
DSC enables the measurements of the transition, such as the glass transition, melting, and crystallization. It also enables the measurement of chemical reactions, such as thermal curing, heat history, specific heat capacity, and purity analysis.
In recent years, there has been a surge in demand for thermal property analysis of highly functional polymeric materials, which has led to a significant increase in the application of DSC.
How Does Temperature Modulated DSC Work?
TM-DSC enables the simultaneous acquisition of heat capacity component data corresponding to specific heat capacity in addition to the information obtained from DSC measurements. The method involves heating a sample at a constant rate through repeated sinusoidal temperature control.
The sample temperature is raised on average at a constant rate through the repeated heating and cooling of the temperature in short intervals (as depicted in Figure 1).
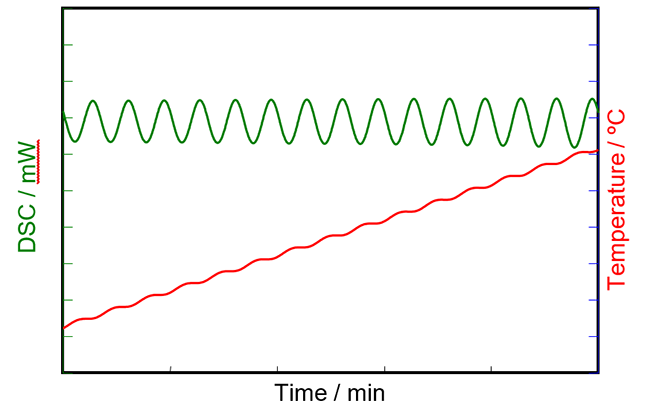
Figure 1. TM-DSC measurement results. Image Credit: Hitachi High-Tech Analytical Science
The data obtained from DSC measurements, which is equivalent to the DSC curve, is referred to as the total heat flow.
The components of the data that are not associated with specific heat capacity can be obtained by subtracting the heat capacity component from the total heat flow, resulting in the data referred to as the kinetic component or non-reversing heat flow.
The Information Provided by TM-DSC
The glass transition causes a change in the specific heat capacity of a material before and after it occurs, which is reflected in the heat capacity component of the DSC signal.
In contrast, peaks that correspond to irreversible phenomena, such as enthalpy changes resulting from thermal curing reactions, cold crystallization, vaporization, or volatilization, appear in the kinetic component of the signal (as summarized in Table 1).
Table 1. Classification of DSC Measurement Phenomena. Source: Hitachi High-Tech Analytical Science
Specific Heat Component |
Kinetic Component |
Glass transition |
Evaporation
Crystallization
Curing
Decomposition, etc. |
The Advantages of Using TM-DSC
TM-DSC offers the advantage of obtaining two separate components, namely, the heat capacity and kinetic components, through a single measurement, which cannot be achieved through conventional methods. This separation is particularly useful when the glass transition and other irreversible phenomena overlap.
In situations where multiple measurements were previously required due to water evaporation or the need to eliminate thermal history, TM-DSC allows for data to be obtained through a single measurement, thereby improving measurement efficiency.
The advantages of using TM-DSC include the following:
- Improved separation of proximities or overlapped transitions
- Improved detection of small transitions and secondary transitions
- Separation into a heat capacity component and kinetic component
- Specific heat capacity measurement (Specifically, specific heat capacity measurement under the low heating rate.)
Measurement Examples with TM-DSC
Figure 2 presents a measurement example of amorphous polyethylene terephthalate (PET) using TM-DSC.
The total heat flow is the same result obtained using conventional DSC. Shifting due to glass transition at 75-82 °C and exothermic peak from cold crystallization at 132 °C are observed in the total heat flow.
The heat capacity component shows the glass transition, while the kinetic component reveals the cold crystallization.
The phenomena measured by DSC can be separated into a heat capacity component and a kinetic component.
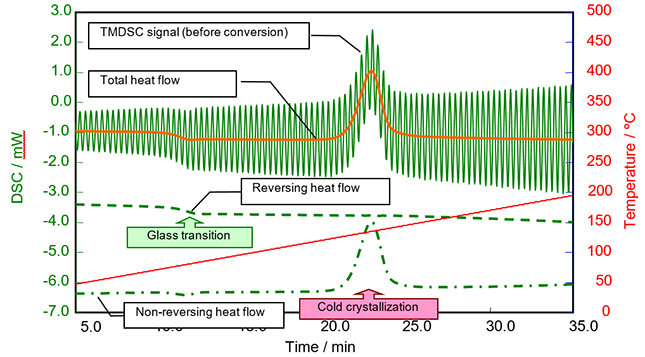
Figure 2. TM-DSC conversion results for amorphous PET. Image Credit: Hitachi High-Tech Analytical Science
The TM-DSC measurement mode and analysis for the separation into a heat capacity component and kinetic component are readily available with Hitachi’s NEXTA DSC. No additional software is required as all the advanced functions are integrated into the NEXTA package software.
More About Hitachi NEXTA DSC Series
The NEXTA DSC Series instruments boast exceptional sensitivity and baseline reproducibility, enabling precise testing and evaluation of even the most minute thermal events.
The DSC200 incorporates cutting-edge technology, making it ideal for a wide range of routine applications with no limitations. The DSC600 is specifically engineered to cater to advanced materials development and failure analysis thanks to its high sensitivity and resolution, which are particularly beneficial in applied research.
The innovative Real View® camera system seamlessly integrates with the NEXTA DSC Series, allowing for real-time observation during measurement.
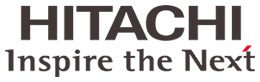
This information has been sourced, reviewed and adapted from materials provided by Hitachi High-Tech Analytical Science.
For more information on this source, please visit Hitachi High-Tech Analytical Science.