The purpose of the exhaust gas analysis is to determine the composition and concentration of the different gases. The analysis is essential, especially for evaluating the air-fuel ratio, surplus air, products of combustion, and unburned constituents. As this is a pivotal factor in environmental preservation and pollution control, it is essential to understand the various techniques of exhaust gas analysis and their applications.
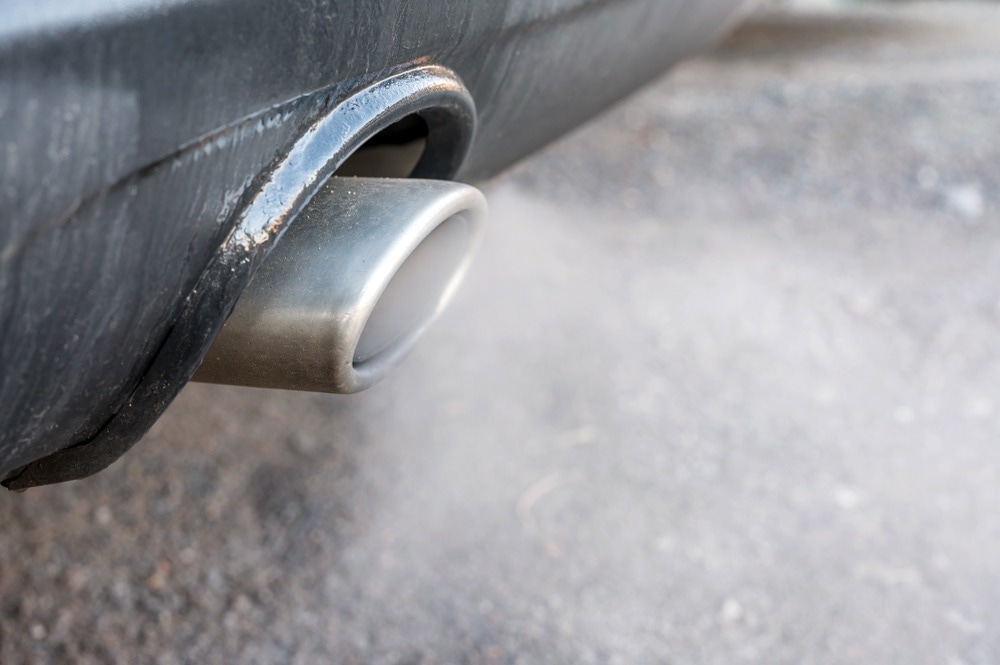
Image Credit: Ralf Geithe/Shutterstock.com
Conventional Orsat Analysis Technique
Orsat analysis is a popular technique for analyzing exhaust gases. It has been employed for decades in numerous industries, including energy production, petroleum refining, and environmental remediation.
The analysis is conducted by pulling an exhaust gas specimen into a burette containing a reagent mixture. The reagent solution reacts with a specific gas component, and the resulting volume change provides a quantitative measurement of the gas concentration.
The precision of the analysis is contingent upon the integrity of the reagent solution, the standardization of the analyzer, and the sampling method employed. Carbon monoxide and nitrogen oxide emissions from combustion operations are particularly amenable to Orsat analysis.
The analysis provides accurate data on the air-to-fuel ratio and combustion temperature, which may be utilized to enhance the efficiency of combustion systems. In short, Orsat analysis is an excellent instrument for exhaust gas analysis.
Utilization of Gas Chromatography for Exhaust Gas Analysis
Gas chromatography is the most extensively used technique for exhaust gas analysis. It is a method for separating the constituents of an exhaust gas mixture per their molecular size and chemical characteristics. The gas chromatograph functions by transferring a specimen of exhaust gas down a column containing a stationary phase, such as silica gel, and a mobile phase, such as helium or nitrogen. Each component of the mixture moves through the column at a distinct velocity, enabling separation and detection.
Non-Dispersive Infrared (NDIR) Spectroscopy
Non-Dispersive Infrared (NDIR) analyzers are the professional standard for determining the carbon monoxide and Carbon dioxide content in exhaust gases.
Each component gas in a sample absorbs infrared radiation at a certain frequency. An NDIR detector may determine the volumetric percentage of CO or CO2 in a sample by projecting an infrared pulse through a specimen cell (carrying CO or CO2) and evaluating the quantity of infrared absorbed by the sample at the required wavelength.
A chopper wheel positioned in front of the detector continuously corrects the analyzer's offset and gain, allowing a single sampling unit to detect the quantities of two distinct gases.
Fourier Transform Infrared (FTIR) Spectroscopy for Exhaust Gas Analysis
FTIR (Fourier transform infrared spectroscopy) is a technique that can detect numerous exhaust chemicals. Many substances take in infrared rays as per their concentration at an inherent wave number (or wavelength).
A proportion of the infrared (IR) energy is captured by the specimen, and the rest is transmitted through it. The ensuing molecular uptake and emission pattern can be employed to determine the sample's constituents and concentration.
In comparison to previous IR approaches, FTIR can measure a greater number of components in real-time by adopting an interferometer that collects a broad spectrum of wavelengths. In contrast, non-dispersive infrared (NDIR) analyzers measure a single chemical due to the employment of an optical filter that enables the selection of a limited wavelength region that is particular to the target component.
How is a Flame Ionization Detector Utilized?
A Flame Ionization Detector (FID) is deployed for this analysis. FID operates on the assumption that H2 air flame includes tiny amounts of ions. Yet, if trace amounts of an organic component such as a hydrocarbon are put into the flame, a high number of ions are generated.
If the polarized voltage is supplied, ion migration will generate a current proportional to the number of ions and, by extension, to the concentrations of exhaust gases present in the flame.
FID output is determined by the number of carbon atoms traversing a flame per unit of time. In other words, the output of FID is proportional to the velocity of carbon atoms in the flame. Oxygen in the exhaust gas may somewhat alter the FID measurement, whereas CO, CO2, water, NO2, and H2 have no effect.
FID analysis is commonly utilized because of its rapid technique and precise determination of the concentration of exhaust gases.
Applications of Exhaust Gas Analysis
Exhaust gas analysis is used to measure the contaminants generated by automobiles, industrial operations, and power plants. The results of the investigation are used to evaluate the adherence to environmental rules and enhance the efficiency of fuel combustion.
What Do the Results of Exhaust Gas Analysis Depict?
The findings of exhaust gas analysis reveal several facts on the chemistry of engine exhaust gases. The major gases include CO, CO2, hydrocarbons, and nitrogenous compounds.
Carbon monoxide (CO) is a toxic gas that results from partial oxidation. The amount of CO in the exhaust gas is an indicator of combustion efficiency.
The greenhouse gas carbon dioxide (CO2) is linked to global warming. The amount of fuel burned during ignition is reflected by the amount of CO2 in the exhaust gas.
Hydrocarbons (HC) are a class of combustion-produced compounds. The quantity of hydrocarbons in the engine exhaust is an indicator of the engine's or ignition process's thoroughness and performance.
Nitrogen oxides (NOx) are a class of gases that contribute to acid rain and smog formation. The concentration of NOx in the exhaust gas is indicative of the engine's or combustion process's contribution to air pollution.
Case Study
In the latest research published in Energies, the exhaust efficiency of heavy-duty trucks employed in the transport of various loads was studied. Two road units were utilized for testing purposes.
Vehicle (A) was a trailer fitted with a stanchion tail. During the test, the truck was transporting logs weighing a total of 25,000 kg. The second vehicle was a curtain-sided tractor unit. Throughout the testing, the truck moved a total of 24,800 kg of steel.
Higher engine load and speed are associated with the greatest emission intensities. On the journey to the forest, the greatest CO2 emission level reached by vehicle (A) was roughly 51 g/s. The maximum CO emission intensity was found over the whole speed range and at the highest values of engine load (800 Nm; 1600 Nm>).
When driving unloaded on the forest roads, small amounts of nitrogen oxides were emitted from the engine in every operating mode. In these regions, the exhaust component's contribution to the emission rate was low, averaging 0.0012 mg/s. These results demonstrate the excellent performance of the catalytic reduction system.
For vehicle B, the average CO2 emission per second was 13.39 grams per second. For every kilometer driven during the test, roughly 1014 grams of exhaust components were emitted into the environment. The maximum intensity of carbon dioxide emissions was almost 39 g/s.
The greatest carbon monoxide emission intensities at full engine load were 127.3 mg/s at 800 rpm and 194.1 mg at 1400 rpm.
For the test run of the heavy-duty vehicle hauling two tons of cargo, the greatest NOx emission intensity of more than 389 mg/s occurred at engine speeds between 1600 and 1800 revolutions per minute and torque between 1200 and 1600 Newton-meters. These results help determine meaningful relationships between vehicle weight, engine rpm, distance, and exhaust emissions.
In short, exhaust gas analysis is necessary for reducing contaminants harming ecological systems. With the advancement of technology and modern equipment, exhaust gas analysis is expected to be much faster and more accurate.
More from AZoM: Flexible Sensor Integration to Bring Valuable Machine Insight
References and Further Reading
Cambustion, 2023. NDIR Principle. [Online]
Available at: https://www.cambustion.com/products/knowledgebase/ndir-principle
[Accessed 23 March 2023].
MRU Instruments, 2023. What is an exhaust gas analyzer?. [Online]
Available at: https://mru-instruments.com/what-does-an-exhaust-gas-analyzer-measure-and-what-are-its-uses/
[Accessed 24 March 2023].
S, Trishna. Measurement of Exhaust Gas Constituents: Conventional and Modern Methods. [Online]
Available at: https://www.engineeringenotes.com/thermal-engineering/fuels-and-combustion/measurement-of-exhaust-gas-constituents-conventional-and-modern-methods-thermodynamics/51333
[Accessed 24 March 2023].
Giechaskiel B, Clairotte M. (2021). Fourier Transform Infrared (FTIR) Spectroscopy for Measurements of Vehicle Exhaust Emissions: A Review. Applied Sciences. 11(16). 7416. Available at: https://doi.org/10.3390/app11167416
Ziółkowski A. et. al. (2022). Analysis of Exhaust Emissions from Heavy-Duty Vehicles on Different Applications. Energies. 15(21). 7886. Available at: https://doi.org/10.3390/en1521788
Disclaimer: The views expressed here are those of the author expressed in their private capacity and do not necessarily represent the views of AZoM.com Limited T/A AZoNetwork the owner and operator of this website. This disclaimer forms part of the Terms and conditions of use of this website.