Interview conducted by Emily MageeJun 9 2023
In this interview, AZoM speaks with Dr. William Mustain, Co-Associate Dean for Research in the Department of Chemical Engineering at the University of South Carolina. He discusses how hydrogen could be the future of green energy, and how the engineering community needs more people who are interested in finding practical solutions.
Please could you introduce yourself and your professional background?
I am Dr. William Mustain from the University of South Carolina. I am also their Co-Associate Dean for Research and a professor in the Department of Chemical Engineering. I am an editor for Applied Catalysis B Environmental and the Chair for the Electrochemical Society Energy Technology Division.
How are the University of Carolina and the College of Engineering and Computing involved in the push for green energy sources?
Approximately 40% of the faculty in the College of Engineering and Computing at the University of South Carolina work in energy. We work on a wide range of things, from electrochemical energy conversion and storage, such as batteries and fuel cells, to thermal processes, such as absorption and nuclear reactors.
We work on combustion and advanced fuel, which comprises everything from hydrogen to ammonia and sustainable aviation fuels. Much of the faculty also works in electrical energy conversion and controls, while another group in the department deals in infrastructure and resiliency.
From a funding perspective, the university has brought in about $100 million in energy-related funding in the past five years, while the college brings in approximately $45 million each year. The last five years have brought in that much energy-related research alone, in addition to all other areas of research. These contracts come from the Department of Energy, the Office of Naval Research, Tesla, Apple, Duracell, General Electric, General Motors, Ford, etc.
We have so much exciting stuff going on across the college. My area of expertise is electrochemical engineering, particularly electric vehicles, as we are researching aspects of energy storage, either in batteries or chemicals like hydrogen, and how to utilize them. This area, at least in my group, has grown steadily since I started as a faculty member 15 years ago, and it has been really rewarding.
It has been interesting to watch things that you would consider very academic taking place in the classroom or the lab, becoming real products or integrated into products. Our relationship with Technetics is going in this direction, too, as we have generated a new part that has gone inside of the water electrolyzers that we are making and testing in our lab.

Image Credit: Shutterstock.com/Audio und werbung
Your recently published white paper ‘Effect of Porous Transport Layer Properties on the Anode Electrode in Anion Exchange Membrane Elextrolyzers’ notes that renewable energy sources have seen a significant reduction in cost in recent years. Could you tell us more about the factors behind this?
Solar and wind energy have seen the most reduction in cost due to materials and manufacturing. For example, until about 2012, the cost of solar panels decreased relatively steadily. Since then, the cost of solar panels has been approximately constant.
However, the efficiency has gone up, meaning the power generation per unit area is more significant than it was before. This results in less sunlight required to produce the same amount of power, or if you have the same amount of sunlight, you can generate more power.
Another improvement has been the manufacturing techniques and how they are constructed and assembled. If a homeowner or a business installs solar, a huge part of the cost is labor, and the labor costs have reduced significantly.
Much of this is driven by solar panels becoming significantly more sophisticated, leading to them being lighter and becoming more modular in terms of their construction. This enables three kilowatts of solar to be installed in your house in an afternoon.
Regarding wind power, science has not changed, but engineering has pushed our ability to make much larger turbine blades than before. If you double the size of the blade, you quadruple the energy you can obtain.
A standard metric is the levelized cost of electricity generation which includes government subsidies. One factor that has helped wind considerably in recent years is the engineering and political side, where there is a will to increase the generated wind power.
What challenges exist regarding moving these sources from niche adoption into broader adoption in the wider public?
A manufacturing base is required to make the volume of panels and wind turbines that you are needed to enable this green energy transition.
However, people have invested in non-green technologies, coal, natural gas, etc. What often happens is that the capital investment that has gone into those is not being wasted.
All our coal power plants in South Carolina will be offline by 2028. That energy must be replaced. Suddenly you have 20% less of your electricity generation, for example, like here in South Carolina for coal. Now there is a massive push for that to be replaced almost exclusively by solar power.
Solar has its promises but also its challenges. The sun shines all day, but people do not turn on their washers and dryers. They want to charge their electric cars immediately when they get home, not let them charge overnight when electricity demand is lower. When the sun shines and the wind blows, it does not necessarily align with when the energy needs to be utilized.
The management of the load is critical, and to some extent, it means we are going to have to rethink how we base-load something. We may have to increase nuclear or change using hydro from a base lead to peak shaving. We also must consider how we are going to store the energy.
The day-to-day variation is one thing, but the seasonal energy consumption variation must be considered. Energy utilization varies seasonally, not just hourly or daily, meaning that we need to store energy long-term.
Batteries have received much attention, but not only is it difficult to make and manage them at very large scales, batteries may not be optimal for very long-duration storage due to self-discharge.
This is where green hydrogen comes in. Hydrogen can be used for long-term energy storage. You can generate hydrogen stored in a container or pipeline for many years. Within this time, a battery would self-discharge and become unusable. Short-term batteries are very good options, but in the longer term, they lose their allure, and it gives hydrogen a significant space to play in.
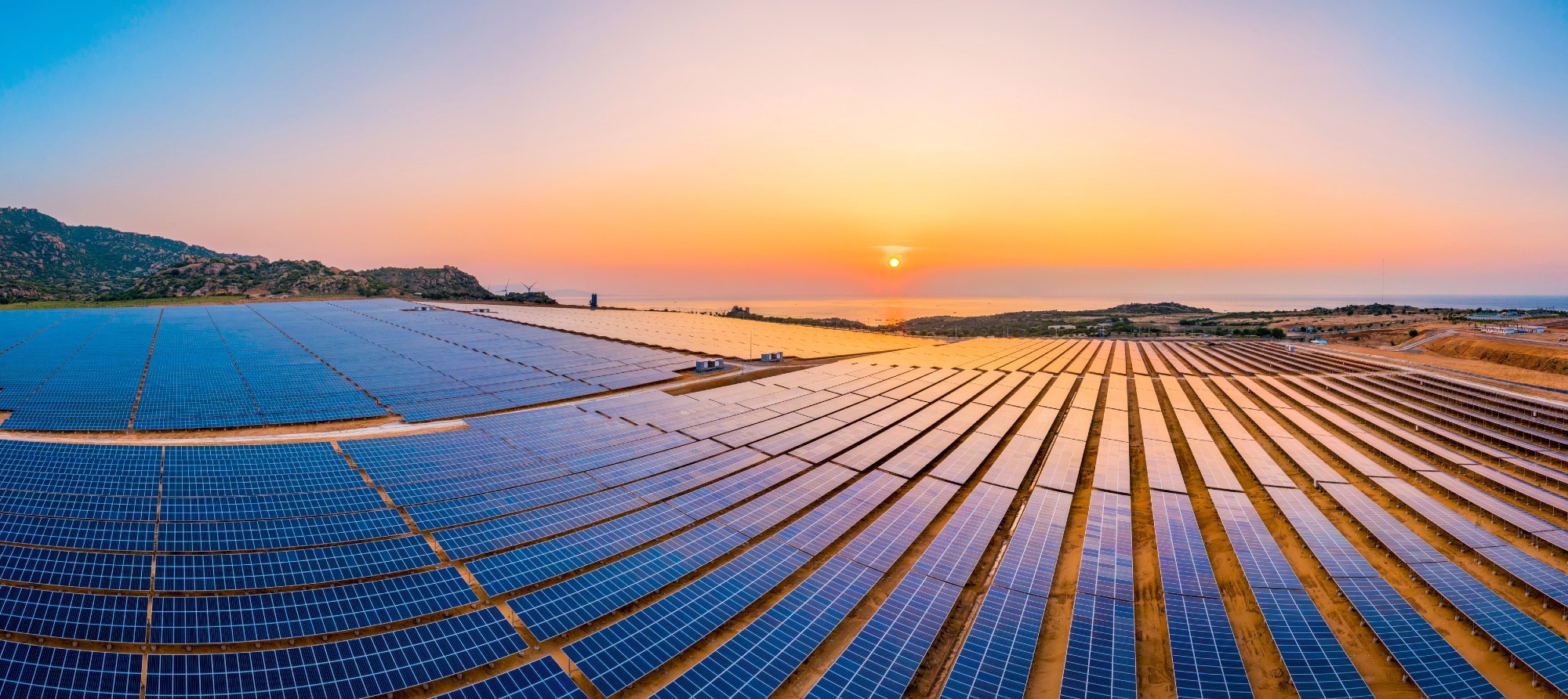
Image Credit: Shutterstock.com/Nguyen Quang Ngoc Tonkin
What challenges are presented by hydrogen?
One challenge is energy density. Hydrogen has the highest gravimetric energy density, a fancy word for per mass. But this is because the hydrogen molecule is extremely small. Per volume, it does not do particularly well. Even if you undergo cryogenic storage, turning it into liquid form, you are at about a third to a quarter of the energy density of diesel, gasoline, or a traditional liquid-based fuel.
Regarding the utilization of hydrogen in transportation applications, energy density is a serious consideration. For example, the volume of your car is not significantly affected by the amount of gasoline you carry.
The gas tank is a reasonable size, and so is a pressurized hydrogen tank. You get about the same range at five kilograms of hydrogen as you do in a tank of gas, and the size of five kilograms compressed is not too unreasonable but would not be reasonable for the volume needed for an airplane or a ship.
The energy density for hydrogen becomes a challenge when you begin trying to mobilize it. More specifically, there are many ways to use hydrogen. For example, consider fuel cells. They are highly efficient, but if you want to use fuel cells, you must completely change the existing fuel infrastructure.
They are also challenging to ramp up and slow down exceptionally quickly. They prefer to be in these hybrid modes, where the fuel cell operates at peak efficiency and the battery does the up and down for you. A gas turbine, for example, does not work this way.
You can ramp up a gas turbine extremely quickly, which is where gas turbines are much better than a solid oxide fuel cell in some applications. However, if you combust hydrogen in a gas turbine, the flame speed is much faster than when using a traditional fuel. Therefore, the turbine design must be changed entirely.
For a hydrogen carrier such as ammonia, the flame speed is so incredibly low it requires blending ammonia with something else to get the turbine to run. Despite hydrogen having serious promise regarding our ability to generate it using renewable energy, it can be a highly reliable energy carrier.
There is also public perception. If you asked people about hydrogen, some people still think about the Hindenburg decades ago, but it has impacted their vision of a hydrogen-fueled future.
Your white paper mentions that anion exchange membrane water electrolyzers have recently received significant attention due to their potential advantages over existing commercial water electrolysis technologies. Could you tell us more about what makes this technology so exciting and some of your findings in the paper?
Currently, alkaline electrolyzer technology overshadows all others. They have been used for over 100 years at some scale but massively scaled for around the last 50 years. Right now, electrolysis only produces about 5% of the world's hydrogen. The other 95% is from steam reforming of fuels, with methane or natural gas being the most common. Although this technology is highly robust and well-studied, it has some problems.
Firstly, you take extremely caustic solutions and flow them throughout a plant where people work, meaning maintenance and safety is an issue. It does not shut the plants down, but people must consider it.
Secondly, the way the cells are designed means that you can only operate at relatively low current and pressure because if higher discharge pressures are reached, the separator between the electrodes is destroyed, resulting in catastrophic failure.
These electrolyzers use cheap nickel materials but are limited in current density and pressure. Around the 1990s, proton exchange membrane electrolyzers became more popular, replacing fragile separators in the middle of the cell with a strong polymer similar to Teflon. This separator shrinks the entire cell.
Instead of having massive systems that operate at low current density, you can make electrodes that are 1,000 times smaller on the micron scale. This means you can get to higher currents because the electrodes are closer to each other and can operate at higher efficiency.
In the traditional alkaline electrolyzer, if you try to blow into a sponge, you blow right through it. However, the separator in the proton exchange membrane electrolyzer is more like a balloon. If you blow on a balloon, it expands. This means it can handle higher pressure, as can the polymer membranes.
This leads to the ability to operate at much higher pressure, which does not require the later compression of hydrogen, saving energy.
The high current means the systems can be small, but the negative side is that they use many expensive materials. Platinum and iridium are the cathodes and the anode materials, with platinum being $1,000 an ounce, while nickel is tens of dollars per gram. This is at least two orders of magnitude difference in cost.
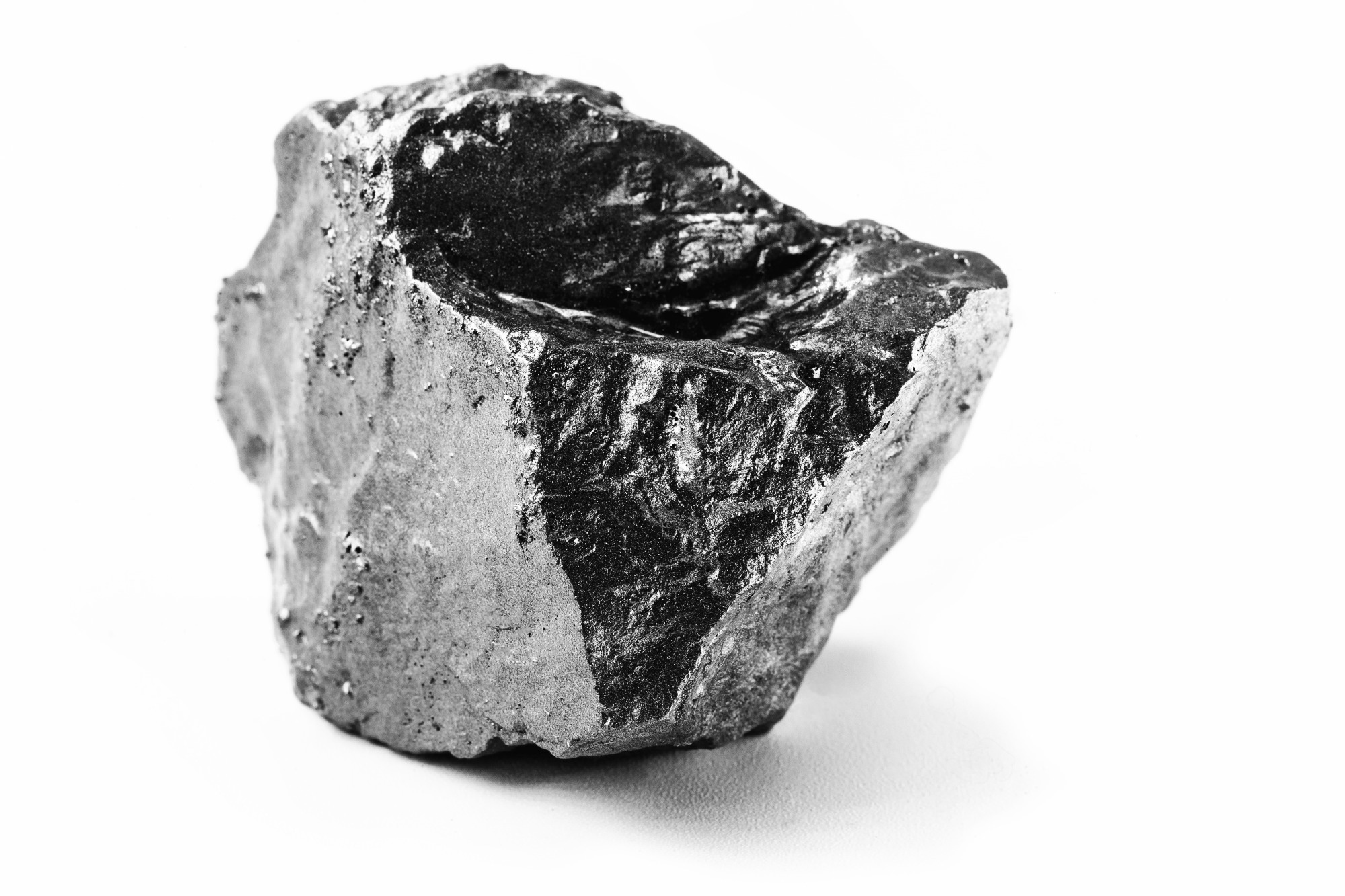
Image Credit: Shutterstock.com/RHJPhotos
The membranes used in the proton exchange membrane electrolyzer are made from fluorinated compounds and PFAs, and in Europe, there is a lot of talk around PFAS and the possibility that it will either be downright banned or so highly regulated that it makes the use of these compounds very costly. This means people are quite convinced that we cannot use those membranes anymore.
Anion exchange membrane fuel cells promise to combine the advantages of these electrolyzers. They have a solid polymer membrane based on hydrocarbons instead of perfluorinated materials, which means it is cheaper and has no PFAS issues. It is also an alkaline environment, so you can probably use lower-cost materials.
A relevant example in our paper was the traditional porous transport layer, which we engineered and designed with Technetics. In the PEM electrolyzer, this is platinum-coated titanium, but in our report, it is a nickel alloy, meaning the cost of the material is significantly lower.
In the rest of the study, we wanted to understand the layer properties, such as density, the alloy material and composition, thickness, and how they impacted the electrolyzer performance.
What does this mean for the public?
I think it means that hydrogen is a little more practical today than it was a year ago or so due to massive investment. Tens of billions of dollars have been announced in Europe, the US, and China.
This investment concerns the alkaline and PEM electrolyzer, but the future is most likely in the anion exchange membrane electrolysis system. Doing anything to bring those to performance at a fraction of the cost will increase the probability of a hydrogen economy 20 or 30 years from now.
Some of us will go to the energy station to fill up with hydrogen, while others will still fill up with gasoline or propane. Some will fill up with electricity, either at the station, at home, or at work. However, if we can bring down the cost of hydrogen, it is much more likely to be used for transportation and energy storage at the grid scale.
The US Department of Energy's goal is $1 per kilogram of hydrogen by the decade's end. A kilogram of hydrogen is about a gallon of gas equivalent, which would be like paying a dollar a gallon again.
Is there anything in your studies that you were not expecting or that stands out as one of the most notable things you have learned?
Most people trying to get funding to work in this area are working on catalysts and membranes because the catalyst is where the reaction happens. The membrane carries the charge and acts as the separator in the cell. Those are the exciting things people think about with water electrolysis or any electrochemical device.
One of my former students, Noor Ul Hassan – who now works at the National Renewable Energy Lab – and I were trying to figure out what the most significant factors are that drive the cell voltage or led to lower cell voltages. We found, inside of our dataset, that the most statistically significant component was the porous transport layer. This was very surprising.
We looked at the most statistically significant component inside the cell if the dataset was constrained. From our statistical analysis, it turned out that it was the porous transport layer.
This study led us to decide that we would start to design the porous transport layer with a partner. We looked for other companies worldwide that made these porous metal meshes. We found that the market is relatively small but growing. We looked at two companies that were established already. In fact, we were already using one as our standard transport layer for a couple of years. That material was quite expensive, and we could not manipulate the properties of the materials at all. As a result, I found the Technetics Group in Columbia, South Carolina, which uses a FELTMETALTM product for a different application.
Our collaborators started to sinter metal particles together with the density and thickness etc. We fine-tuned the control over what we were able to produce and then use inside of the cell. With them, we were able to dial in the size, thickness, density and material for a high-performing PTL.
What principles guide and help form strong partnerships that allow you to work with a company like Technetics in a way that leads you to more successful outcomes?
One of the things that I find in every successful industrial collaboration is that both sides have a vested interest in what you are doing and the tasks inside of the project.
The best collaborations are where you exchange information and work closely. John and Elaine, who are also on the paper, have highly supported what we wanted to do.
We are a very engineering-oriented group and have a vested interest in finding a transport layer that works. From the side of Technetics, it was a fascinating, intellectual problem and something that would be a commercializable product in the end. It was a win-win, and I think it continues to be.
Do you have any final thoughts to add?
There are many ways to get involved in this green hydrogen push. We require people to generate the energy and electricity we need to store for days and seasons. We need people to work on hydrogen storage and high-pressure seals and to build electrolyzers and components.
Whether you decide to be a chemical, mechanical, electrical, computer, or civil engineer, each will play a role in the hydrogen economy. We have a huge shortfall of engineers in the country, and if something like this sounds exciting to you or someone you know, contact me. I will talk to them because we certainly are going to need the workforce to do this.
I want to highlight that industry-university partnerships can be extremely fruitful. Academics like myself do not just teach in the classroom. There are lots of us that want to help solve real practical problems. If a company has a question that would be great to answer but they do not have the bandwidth, a university is a fantastic way to do it.
Lastly, think about the University of South Carolina. We have a huge energy focus here in the College of Engineering and Computing, and we are carrying out cutting-edge research and $100 million of grants in the last five years related to various energy projects. There is a lot of opportunity here. If anybody wants to get more information, please feel free to reach out and ask me for it. I would be happy to facilitate this for you.
Is there a way for people to contact you with questions?
Emailing me at [email protected] is a great place to start. I also have my website, mustainlab.com. If you are interested in more of our projects and our papers, links to all the papers can be found on the website, including the one that we have with Technetics.
About Dr. William E. Mustain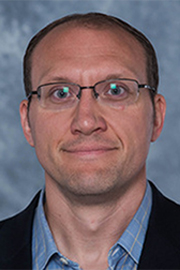
Dr. Mustain is currently the Co-Associate Dean for Research and has been a Professor in the Department of Chemical Engineering at the University of South Carolina since 2017. With over 130 publications in his name, he is passionate about building an internationally recognized research program in electrochemical energy conversion and storage, as well as preparing the next generation workforce by graduating top-notch students that are well trained.
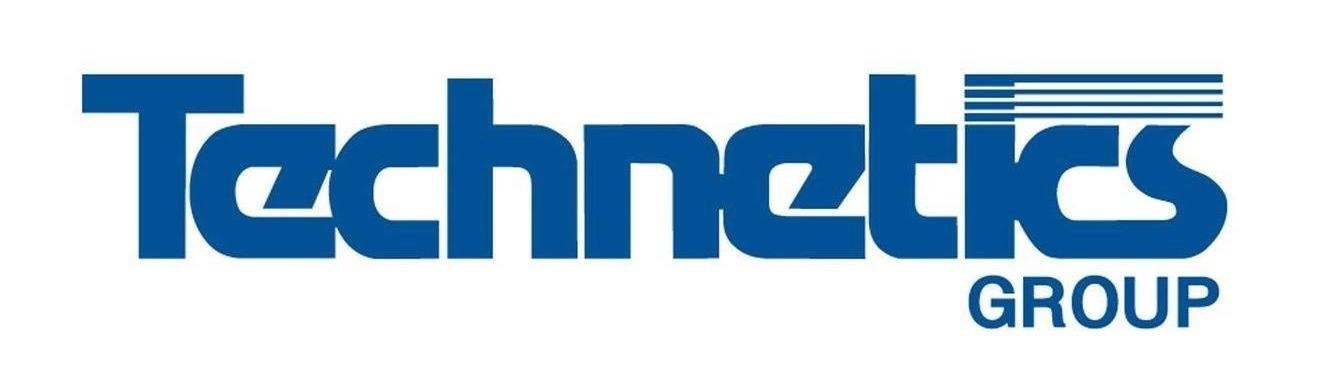
This information has been sourced, reviewed and adapted from materials provided by Technetics Group.
For more information on this source, please visit Technetics Group.
Disclaimer: The views expressed here are those of the interviewee and do not necessarily represent the views of AZoM.com Limited (T/A) AZoNetwork, the owner and operator of this website. This disclaimer forms part of the Terms and Conditions of use of this website.