Redox flow batteries (RFBs) are considered to be technology with the potential to revolutionize large-scale energy storage applications. With their long lifetimes, high energy densities, and scalability to meet varying storage needs, RFBs offer several advantages over other battery technologies.
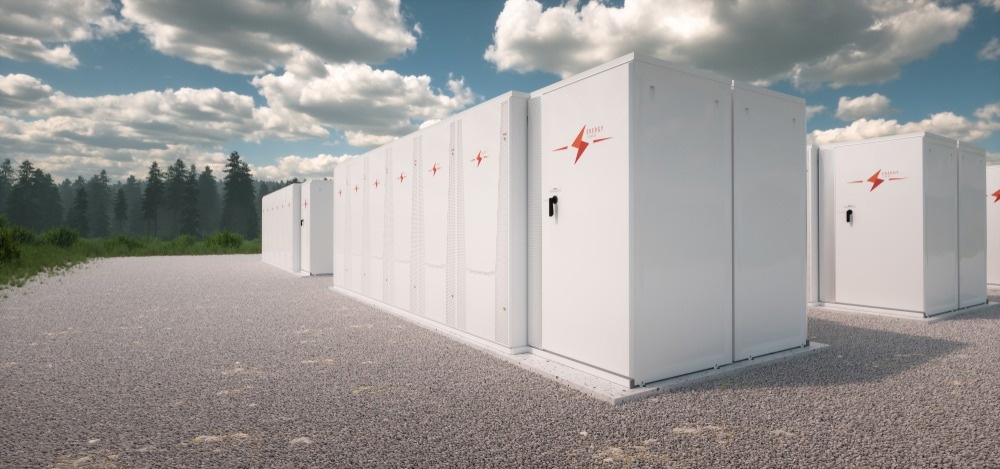
Image Credit: petrmalinak/Shutterstock.com
In this article, we explore the recent progress, exciting developments, and projects in the field of redox flow batteries. These advancements propel RFBs as a competitive option for large-scale energy storage and contribute to the global transition towards a greener and more sustainable energy future.
Addressing Challenges and Recent Developments in Redox Flow Batteries
Despite their advantages, RFBs face challenges that necessitate ongoing research and innovation. Researchers are currently exploring ways to improve battery performance and address critical research gaps.
Recent advancements in RFB technology have shown promise in areas such as electrolyte solutions, electrode materials, cell designs, and manufacturing processes.
One notable breakthrough is the development of new electrolyte solutions that offer increased stability and longer lifespans. These advanced solutions enhance the overall performance and efficiency of RFBs, making them more competitive with other battery technologies. Moreover, researchers have successfully developed new electrode materials that are more efficient and have extended lifespans, further contributing to the improved performance of RFBs.
Cell design plays a crucial role in optimizing battery efficiency and reducing costs. Ongoing research projects focus on developing new cell designs that are even more efficient and cost-effective, making RFBs increasingly attractive for large-scale energy storage applications.
Additionally, efforts are underway to improve manufacturing processes, streamlining production and ensuring higher quality batteries are available for deployment.
In a study published in the Energy Storage Materials Journal, researchers discuss a groundbreaking membrane-free, aqueous/nonaqueous hybrid redox flow battery. This battery demonstrated impressive capacity retention of 94.5% over 190 cycles at a current density of 1.0 C.
The research team employed cutting-edge techniques such as density functional theory (DFT) calculations and operando spectroscopies to gain insights into minor side reactions and monitor electrolyte water content. This breakthrough contributes to cost-effective energy storage and opens avenues for detailed mechanistic studies and practical applications of redox flow batteries.
Research and Development Support to Develop Redox Flow Batteries
The United States Department of Energy (DOE) has recognized the potential of energy storage technologies and has committed substantial funding to advance their development.
In March 2023, the Energy Storage Demonstration and Validation FOA announced $27 million in funding, including $12 million for research, development, and demonstration projects focused on the commercial development of grid-scale lithium and redox-flow batteries. This support underscores the importance of RFBs in the pursuit of large-scale energy storage solutions.
Techno-Economic Analysis for Sustainable Chemistries in Redox Flow Batteries
Techno-economic analysis plays a vital role in assessing the economic viability of different battery chemistries.
Researchers at the Massachusetts Institute of Technology (MIT) have developed a modeling framework to accelerate the development of flow batteries for large-scale, long-duration energy storage on the grid. MIT researchers are exploring alternative chemistries that utilize more abundant and cost-effective materials, reducing dependency on limited and volatile resources like vanadium.
By considering operating challenges early in the design process and pursuing cost-effective chemistries, they aim to make flow batteries economically viable for widespread adoption.
Recent Pilot Project and Deployments of Redox Flow Batteries
Pilot projects and commercial deployments further showcase the potential of RFBs for large-scale energy storage. For instance, energy company RWE is working on the Panta.rhei redox flow pilot project, which involves 30 redox flow modules with a total capacity of 130 kW.
Such projects will help to demonstrate the practical application of RFBs and their ability to store green power, providing a crucial solution for balancing intermittent renewable energy generation.
Conclusions and Perspectives
Advancements in redox flow batteries hold great promise for large-scale energy storage applications. Ongoing research and development efforts focused on improving electrolyte solutions, electrode materials, cell designs, and manufacturing processes are driving the competitiveness and cost-effectiveness of RFBs.
Looking ahead, the global energy storage market is projected to experience substantial growth. According to the DOE, By 2030, the combined stationary and transportation energy storage markets could expand up to five times their current capacity, reaching 2.5-4 terawatt-hours (TWh) annually.
Electrified powertrains are anticipated to drive energy storage demand, surpassing stationary applications in energy capacity usage.
This growth is fueled by developments in electrified transportation, decreasing battery costs, and increased renewable generation. Notably, stationary energy storage is now seen as an opportunity to enhance grid reliability and resilience, leading to significant market growth in regions such as North America, China, and Europe.
The market for redox flow batteries is also poised for growth. According to Market Watch, the global Redox Flow Battery market was valued at USD 2084.69 million in 2022 and is expected to reach USD 2145.85 million by 2028, expanding at a compound annual growth rate of 0.48%. These figures reflect the increasing adoption and recognition of RFBs as a viable solution for large-scale energy storage.
As the global energy storage market grows and evolves, RFBs are poised to play a vital role in enhancing grid reliability, balancing renewable energy generation, and supporting the transition to a sustainable energy future.
More from AZoM: Alternative Electrode Materials for High-Performance Batteries
References and Further Reading
Critchley, L. (2017). An Overview into Redox Flow Batteries [Online]. AZoM.com. URL https://www.azom.com/article.aspx?ArticleID=14877 (accessed 6.29.23).
DOE (2020). Energy Storage Grand Challenge: Energy Storage Market Report (No. NREL/TP--5400-78461, 1908714, MainId:32378). https://doi.org/10.2172/1908714
Marketwatch (2023). 2023 “Redox Flow Battery Market” Research | With 106+ Pages [Online]. MarketWatch.com. URL https://www.marketwatch.com/press-release/2023-redox-flow-battery-market-research-with-106-pages-2023-06-16 (accessed 6.29.23)
Office of Electricity (2023). U.S. Department of Energy Announces $27 Million To Advance Energy Storage Technologies [Online]. Energy.gov. URL https://www.energy.gov/oe/articles/us-department-energy-announces-27-million-advance-energy-storage-technologies (accessed 6.29.23).
RWE (2023). Everything flows” – RedOx flow pilot project panta.rhei at the RWE Campus [Online]. rwe.com. URL https://www.rwe.com/en/research-and-development/battery-storage-system-projects/redox-flow/ (accessed 6.29.23).
Schubert, C., Hassen, W.F., Poisl, B., Seitz, S., Schubert, J., Usabiaga, E.O., Gaudo, P.M., Pettinger, K.-H. (2023). Hybrid Energy Storage Systems Based on Redox-Flow Batteries: Recent Developments, Challenges, and Future Perspectives. Batteries 9, 211. https://doi.org/10.3390/batteries9040211
Stauffer, N. (2023). Flow batteries for grid-scale energy storage [Online]. energy.mit.edu. URL https://energy.mit.edu/news/flow-batteries-for-grid-scale-energy-storage/ (accessed 6.29.23).
Taylor-Smith, K. (2021). Recent Developments and the Future of Redox Flow Batteries [Online]. AZoM.com. URL https://www.azom.com/article.aspx?ArticleID=20531 (accessed 6.29.23).
Wang, X., Lashgari, A., Chai, J., Jiang, J. “Jimmy,” (2022). A membrane-free, aqueous/nonaqueous hybrid redox flow battery. Energy Storage Materials 45, 1100–1108. https://doi.org/10.1016/j.ensm.2021.11.008
Disclaimer: The views expressed here are those of the author expressed in their private capacity and do not necessarily represent the views of AZoM.com Limited T/A AZoNetwork the owner and operator of this website. This disclaimer forms part of the Terms and conditions of use of this website.