Material engineering involves the creation and design of inventive materials, blending principles from chemistry, physics, and engineering. Mass spectrometry emerges as a vital tool in material engineering, offering unprecedented insights into surface chemistry intricacies. This article delves into the expanding importance of mass spectrometry in reshaping surface chemistry and its pivotal role in advancing material synthesis.
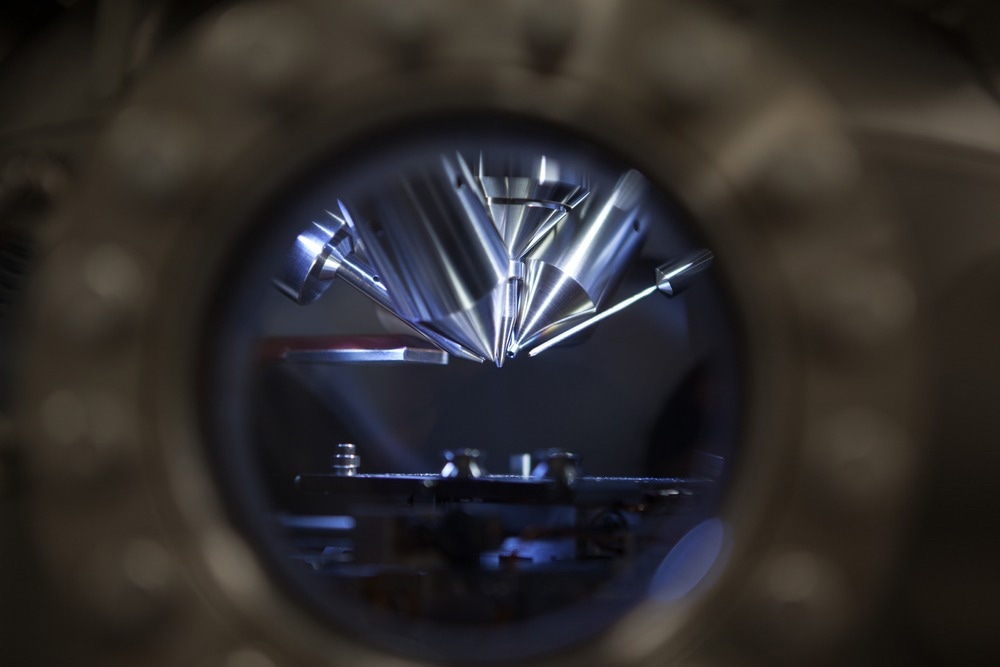
Image Credit: Intothelight Photography/Shutterstock.com
Surface chemistry critically influences material properties and performance, as the outermost layer governs interactions with the environment. Mass spectrometry, a potent analytical technique, identifies and quantifies chemical compounds based on mass-to-charge ratio by ionizing a sample, separating resulting ions by mass, and detecting them to generate a mass spectrum.
In mass spectrometry, a primary ion beam directed onto the sample's surface collides with surface atoms, causing the ejection of secondary ions. These ions are accelerated into a mass spectrometer and sorted based on the mass-to-charge ratio (m/z), allowing the identification of elements and compounds present on the surface.
Significance of Mass Spectrometry in Materials Science and Synthesis
Mass spectrometry (MS) is pivotal in materials science and synthesis, offering crucial information about material composition, structure, and properties. It identifies and quantifies elements and compounds, providing insights into overall composition. For example, it is widely used in polymer analysis to determine molecular weights, polymer structures, and the presence of additives.
Similarly, mass spectrometry is a powerful tool for quality control during the synthesis of materials, allowing researchers to monitor and verify the composition of synthesized materials, ensuring that they meet the desired specifications and have the required properties.
Combination with Other Techniques
Mass spectrometry can be combined with other analytical techniques, such as chromatography and spectroscopy, to provide comprehensive information about materials. This multi-dimensional approach enhances the accuracy and depth of analysis. For instance, in 2016, researchers explored the revolutionary potential of combining high-resolution scanning probe microscopy (SPM) with preparative mass spectrometry (pMS) for advancing material engineering at the atomic scale.
The researchers demonstrated the application of preparative mass spectrometry technology, involving controlled deposition of ion beams, for achieving atomic-scale chemical control of molecular deposition in ultrahigh vacuum. This approach allowed for investigating large molecules with SPM, providing insights into their structure, properties, and electronic behavior. The study emphasized the role of preparative mass spectrometry in offering a platform for novel molecular materials research, paving the way for advancements in nanotechnology and surface science.
Recent Developments
In a recent study, researchers have highlighted the revolutionary role of mass spectrometry in material engineering, particularly in the field of surface chemistry. The study underscores recent developments and notable advancements, including ion mobility and tandem mass spectrometry to reveal structural details and the development of new hyphenated techniques for finer structural analysis. The integration of mass spectrometry with spectroscopic tools further enhances the characterization of materials, marking a significant stride towards mass spectrometry evolving into a universal tool for material characterization.
Understanding Surface Chemistry of Lignocellulosic Biomass via ToF-SIMS
In another development, researchers explored the application of Time-of-Flight Secondary Ion Mass Spectrometry (ToF-SIMS) in understanding the surface chemistry of lignocellulosic biomass, crucial for efficient biomass conversion into biofuels. ToF-SIMS allows spectral and spatial analysis, revealing insights into biomass surface chemistry impacted by chemical pretreatments, microbial treatments, and genetic modifications. Recent advances include 3D molecular imaging, where researchers stacked ToF-SIMS images to provide a comprehensive view.
The study emphasizes ToF-SIMS' role in characterizing biomass at the molecular level, addressing instrument principles, biomass sample preparation, and lignocellulosic ion fragmentation peaks. Notably, the technique aids in comparing genetic modifications, assessing enzymatic hydrolysis efficiency, and studying the impact of various pretreatments on biomass surface chemistry, offering valuable insights for material engineering in biofuel production.
Challenges and Future Prospects
Despite significant advancements in material engineering facilitated by mass spectrometry, there are still challenges that need to be addressed. For instance, the sensitivity of mass spectrometry to sample conditions, such as vacuum requirements in traditional methods, can limit its applicability to certain materials. However, advancements in ambient mass spectrometry techniques are addressing these limitations, allowing for the analysis of materials under near-ambient conditions.
The future of mass spectrometry in material engineering holds exciting prospects. Continued innovation in instrument design, data analysis algorithms, and sample preparation techniques will likely further expand the capabilities of mass spectrometry in surface chemistry. Additionally, interdisciplinary collaborations between chemists, physicists, and materials scientists can play a crucial role in unlocking the full potential of mass spectrometry for materials research.
Conclusion
In conclusion, mass spectrometry stands as a transformative force in material engineering, particularly in revolutionizing surface chemistry. Its pivotal role in analyzing surface composition, identifying compounds, and ensuring quality control in material synthesis underscores its significance in materials science.
Recent developments, including advancements in ion mobility and tandem mass spectrometry, showcase its evolving potential. Despite challenges, ongoing innovations, interdisciplinary collaborations, and integration with other techniques promise a future where mass spectrometry continues to be a universal and indispensable tool, driving advancements in nanotechnology, surface science, and beyond.
More from AZoM: How are Bioplastics Made?
References and Further Reading
Chakraborty, P., & Pradeep, T. (2019). The emerging interface of mass spectrometry with materials. NPG Asia Materials. https://doi.org/10.1038/s41427-019-0149-3
Ingle, Rebecca. (2023, January 18). What is Mass Spectrometry?. AZoOptics. Retrieved on November 17, 2023 from https://www.azooptics.com/Article.aspx?ArticleID=2349.
Meštrović, Tomislav. (2023, July 19). What is Mass Spectrometry?. News-Medical. Retrieved on November 17, 2023 from https://www.news-medical.net/life-sciences/What-is-Mass-Spectrometry.aspx.
Rauschenbach, S., Ternes, M., Harnau, L., & Kern, K. (2016). Mass spectrometry as a preparative tool for the surface science of large molecules. Annual Review of Analytical Chemistry. https://doi.org/10.1146/annurev-anchem-071015-041633
Tolbert, A., & Ragauskas, A. J. (2017). Advances in understanding the surface chemistry of lignocellulosic biomass via time‐of‐flight secondary ion mass spectrometry. Energy Science & Engineering. https://doi.org/10.1002/ese3.14
Disclaimer: The views expressed here are those of the author expressed in their private capacity and do not necessarily represent the views of AZoM.com Limited T/A AZoNetwork the owner and operator of this website. This disclaimer forms part of the Terms and conditions of use of this website.