The medical device sterilization industry has faced increasing scrutiny over the past 10 years, due to a series of sentinel events and resulting personal injury suits surrounding its use of the sterilant gas ethylene oxide (EtO).
These events, and the resulting regulations to prevent them from occurring again, have significantly pressured sterilization facilities to adopt the most advanced monitoring and control strategies available to ensure regulatory compliance and limit their liability moving forward.
Companies seeking to adhere to these new rules must limit worker and community exposure to EtO via a range of facility enhancements while ensuring the continuous flow of critical goods through the medical device supply chain.
Particular challenges have arisen around the need to ensure effective EtO abatement in problem areas, such as laboratories and office spaces on discrete HVAC circuits and warehouse areas accommodating freestanding finished goods.
This article explores the performance of Sonata Scientific’s Helios EtO control technology platform - a novel, low-power, photocatalyst-based technology for abating EtO in these problem settings.
The platform’s performance is validated via a number of case studies, using the proven capabilities of Picarro’s EtO Workplace Monitoring System (WMS).
Extensive real-world EtO datasets from participating sterilizer facilities equipped with Picarro’s WMS are examined, with these real-world conditions then simulated in laboratory settings to evaluate the effectiveness of Sonata Scientific’s Helios solution.
Particular focus is placed on reducing EtO to levels below 10 ppb, the target level proposed in the original announcement of the FIFRA rule for EtO workplace exposure.
These case studies confirm that Helios achieves destruction and removal efficiency (DREs) rates of 98% and above (typically 99%+), even when the air matrix is complicated by carbon dioxide, water vapor, methane, and a range of common cleaning agents.
The Picarro and Sonata Scientific systems’ ground-breaking performance is evidence of a wider, next-generation technological revolution taking place in terms of the measurement and abatement of hazardous chemicals like EtO in the workplace.
The specific products described in this article have the potential to empower facilities to better understand and control facility EtO levels, as they work toward their key goal of improving human health - a goal that is wholly consistent with the significant value that EtO sterilization brings to the medical supply chain.
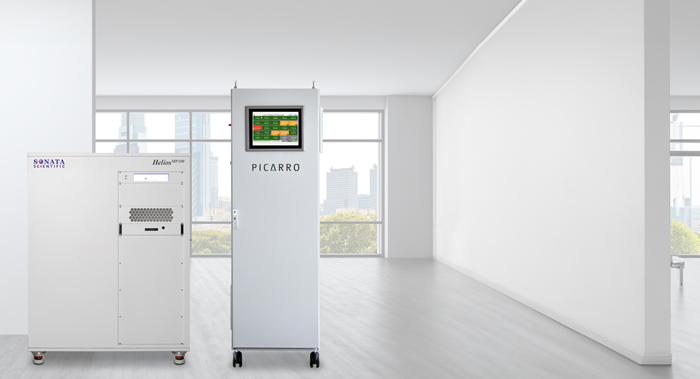
Figure 1. Sonata Scientific HeliosTM HP100 (left) and the Picarro Workplace Monitoring System (right) empower facilities to fundamentally better understand and control their facility EtO levels. Image Credit: Picarro
List of contents
EtO Abatement Strategies
Methods
The Helios Technology Platform
Picarro WMS: A CRDS Solution
Three Different Workplace Scenarios
Workplace Setting One: Trapped and Permeating EtO at High Concentrations
Workplace Setting Two: Warehouse Areas near Bay Doors
Workplace Setting Three: Complex Air Matrices, Laboratory Settings
Conclusions
References and Further Reading
EtO Abatement Strategies
Industrial engineers and hygienists have been working toward effectively ablating EtO in commercial sterilizer facilities for decades. EtO sterilization was initially developed in the 1940s by the US military, and this was refined in the 1950s when the McDonald process was developed to enable the sterilization of medical devices using dedicated sterilization chambers.1
The McDonald process and similar strategies focus on the containment and passivation of EtO during sterilization. In cases where EtO is beyond safe exposure limits and notably above its lower explosive limit, negative-pressure-regulated sterilizer chambers and inert balance gases such as carbon dioxide, nitrogen, and chlorofluorocarbons can address this.
Not only do sterilizer chambers allow for containment, they also enable the rapid mechanical abatement of any EtO present via air flushes. These flushes originally sent the process gas to stack flares or vents, with systems evolving to transport this gas to thermal oxidizers, wet scrubbers, and catalytic oxidizers.
Primary concerns centered around explosion and immediate toxicity when these early sterilization processes were introduced.
As evidence increased confirming the risk to human health at lower EtO levels, further control mechanisms were established to limit worker exposure and facility concentrations of EtO to single-digit part-per-million (ppm) levels. These mechanisms included aeration chambers, dry bed fugitive emissions scrubbers, and back-vent interlocks.
Aeration chambers house recently sterilized finished goods for between 8 hours and several days because this is when the highest levels of EtO off-gassing take place.
Dry beds can pull significant gas volumes through scrubbing media at room temperature. These work by either scrubbing EtO from process gas as it is transported to the stack, or by scrubbing EtO from ambient air before recirculating this back into the facility, effectively minimizing the need to condition fresh outdoor air.
Extra back-vents, or ‘chamber exhaust vents,’ can be added with chamber door interlocks to reduce worker exposure during chamber unloading. These work by pulling ambient air into the chamber and venting it to another scrubbing mechanism or to the atmosphere.
These methods have improved the health and safety of workers and neighboring communities, but new and updated US and international regulations increasingly require facilities to lower stack emissions and workplace EtO levels. These regulations include the recently published 2024 EtO NESHAP revision (40 CFR Pt 63 Subpart O)2 and FIFRA PID.3
EtO’s unique attributes make the comprehensive reduction of its fugitive emissions complex. For example, EtO does not predictably exit a facility like CO2, which engineers use to gauge a facility’s air turnover and dispersion.
Rather, EtO permeates building materials, continuing to off-gas from products for a long time after the completion of sterilization and even aeration.
Data from warehouse quarantine areas (Figure 2, Area (2)) highlight the occurrence of this phenomenon outside a permanent total enclosure (PTE). Here, a product sterilized several days prior can still prompt the rise of ambient EtO to double-digit ppms if there is no system of fugitive emissions abatement in place.
HVAC systems in office (1) and laboratory (3) spaces typically recirculate a large portion of moved air to minimize the need to cool or heat incoming air. However, this process traps fugitive EtO that would have been abated within a PTE.
It is also important to note that building materials do not always isolate EtO within PTEs, because they can be just as permeable to the gas as medical equipment sterilized with EtO.
Many legacy analyzer technologies show significant false positive levels of EtO. This is due to spiking from common chemicals like isopropanol (IPA) and water vapor. This complicates the ability to achieve low part-per-billion (ppb) levels of EtO in facilities, prompting facility supervisors to doubt the accuracy of readings from these legacy devices.
This article uses real-world datasets acquired at participating sterilizer facilities with the Picarro WMS to clarify these dynamics. The Picarro WMS system is highly resilient to common interferences, and able to measure EtO at the ultra-low ppb levels required by FIFRA.
This information is used to evaluate the Sonata Scientific Helios platform in laboratory settings that closely simulate these real-world conditions. This allows a robust assessment of the Helios platform’s ability to abate EtO at ppb to ppm levels.
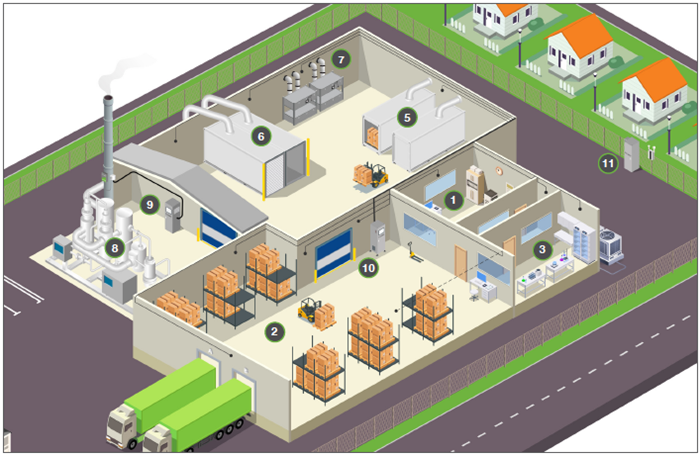
Figure 2. Schematic illustrating the typical areas of an EtO sterilization facility as used today. A PTE area is isolated at the top from the other areas by blue rollup doors, housing sterilization chambers (5), aeration cells (6) and dry beds (7) in between. A CatOx (8) is seen at the far left connecting to the stack which accommodates a Picarro CEMS (9). The Picarro WMS (10) sits in the middle of the image with black lines radiating out to sampling points, while the Picarro fenceline system (11) is also visible on the far right near the houses. The three workplace settings discussed in this paper are annotated as (1), Office areas with HVAC; (2), warehouses near bay doors; and (3), laboratory spaces, or otherwise complex matrices. Image Credit: Picarro
Methods
The Helios Technology Platform
The Helios product line from Sonata Scientific has been designed to abate small-molecule VOCs in low-power simple form factors. The VOCs include low-boiling-point molecules such as EtO, acetaldehyde, formaldehyde, and isopropyl alcohol (IPA).
The powerful EtO control technology found in Sonata Scientific’s Helios product line uses a proprietary catalyst combined with an engineered photoreactor to drive sustainable and high EtO DRE at levels that are consistent with indoor fugitive EtO emissions across a diverse array of environmental conditions.
The Helios platform leverages light rather than heat to continuously convert EtO to CO2 and H2O. This innovative approach allows it to achieve critical illumination levels in minutes without the need for extended start-up sequences.
High performance is ensured by delivering light and EtO-laden air to the active material.
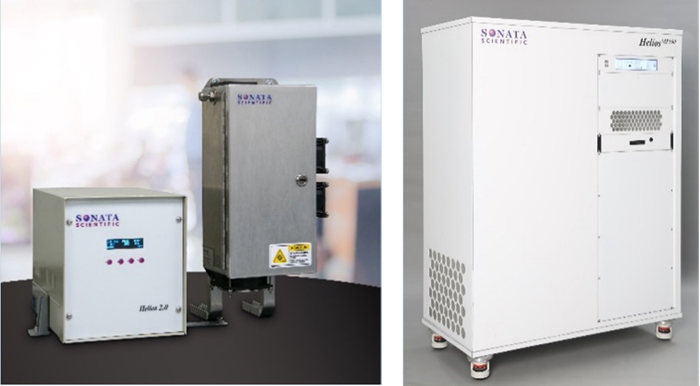
Figure 3. Sonata Scientific Helios technology platforms. (Left) Laboratory-scale Helios 2.0 for small-scale demonstrations and (right) Helios MP100 for on-site facility evaluations. Image Credit: Picarro
The Helios technology platform includes several laboratory-scale reactors (0.5-20 SLM) designed to demonstrate DRE performance under real-world test conditions (Figure 3, left). It also includes higher flow systems (100 CFM and greater) suitable for the majority of on-site sterilizer applications (Figure 3, right).
Many companies struggle to abate EtO at the stack and in the workplace. Sonata’s EtO control systems are anticipated to be central to the abatement process, operating as standalone solutions currently and as key components of integrated industrial abatement systems in the near future.
Picarro WMS: A CRDS Solution
Cavity ring-down spectrometer (CRDS) systems from Picarro offer stable, selective, sensitive, and simple measurements of trace gas EtO. These systems are ideally suited to industrial monitoring applications, such as workplace, fenceline, CEMS, and mobile systems.
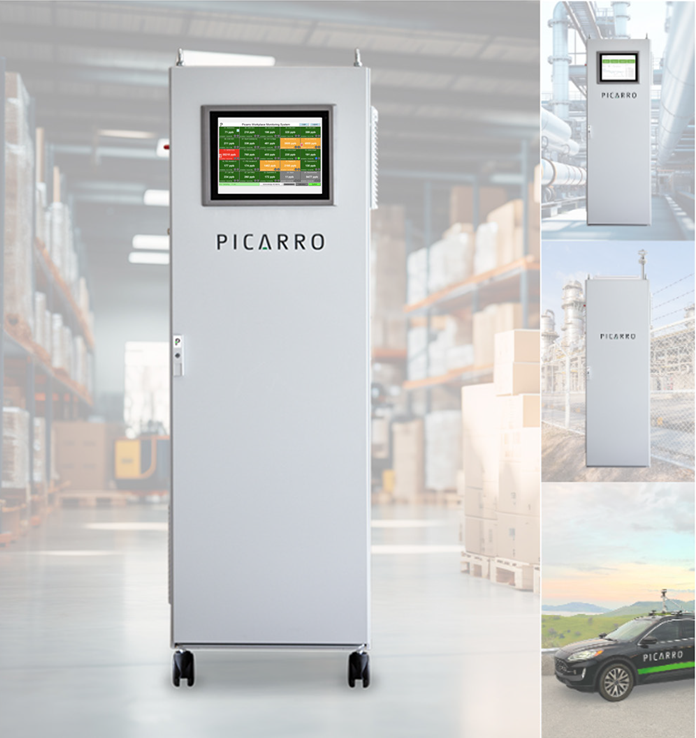
Figure 4. Picarro Workplace Monitoring System (left) with inset images of CEMS, Fenceline, and Mobile systems (top to bottom). Image Credit: Picarro
The Picarro Workplace Monitoring System outlined in this article can sample from up to 25 points across a facility. A typical measurement cycle will acquire data at each position over 24 seconds, enabling complete characterization of a facility within 10 minutes.
The WMS boasts a user-friendly touch-screen interface and is mounted on heavy-duty casters for ease of movement. The instrument consumes ~400 W and ensures continuity via an integrated uninterruptible power supply (UPS).
These features mean that the WMS can be rolled into place, plugged into the simplest 120V or 250V service, connected to lines, and running in under an hour.
The WMS accurately characterizes EtO from single-digit ppb to over 100 ppm. Single, averaged, auto-calibrated values are reported at each position, and any potential issues can be mitigated via flagging for data quality, flow errors, high, and high-high alarms.
The WMS’s core instrument offers high sensitivity and dynamic range, allowing extremely accurate assessment of DRE for abatement systems such as the Helios.
In commercial sterilizer settings, outlet concentrations can range from double-digit ppbs to double-digit ppm, while inlet concentrations can be in the single-digit ppb range or even lower.
The real-world data shown in Figure 5 (acquired from a participating commercial sterilizer partner) demonstrates the power of this technology.
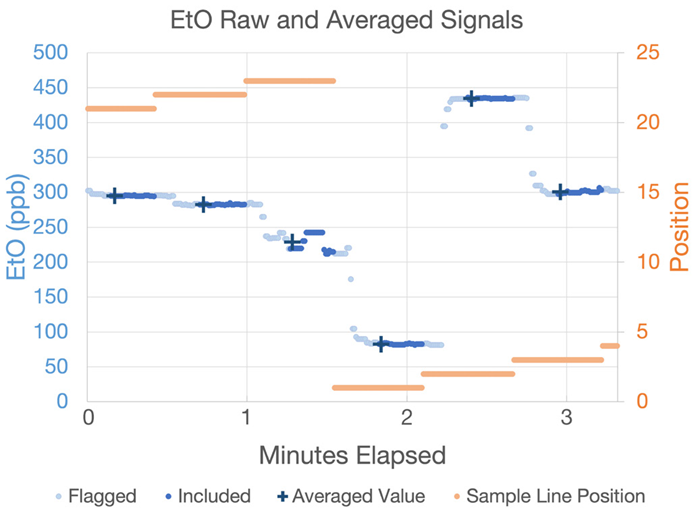
Figure 5. WMS real-time (dots) and averaged (crosses) data shown against sample position (orange dots). Image Credit: Picarro
This example features data collected across multiple positions to showcase the averaging algorithm’s resilience to bias.
Light blue dots mark dead time transition data, while darker blue dots show the data points used to calculate the average. Computed averages are displayed as dark blue crosses time-stamped to the beginning of the averaging period.
The averages calculated at positions 1 and 2 clearly show that transitional data between samples (T90<5 seconds) is not averaged into the final value. This confirms that the calculated averages remain unbiased by the prior sample.
The raw data also shows that some areas have clear homogenous sampling atmospheres (for example, those sampled on positions 21 and 2), while areas like position 23 show evidence of dynamic processes at the sample point, such as the movement of a recently sterilized product.
Workplace monitoring systems from Picarro are currently deployed at sterilizer facilities globally, and the company’s employees have spent many cumulative months with facilities’ staff to thoroughly understand and characterize the complexity of EtO’s movement around their facilities.
These systems are routinely employed to support customers in making key decisions about facility-wide abatement system upgrades and potential capital expenditures.
Three Different Workplace Scenarios
This article discusses three different workplace environments where challenges have been encountered in abatement of EtO with traditional approaches like dry beds and CatOx/RTOs. Picarro has noted these three ‘problem areas’ across a wide range of facilities, whether traditional or modernized, small or large.
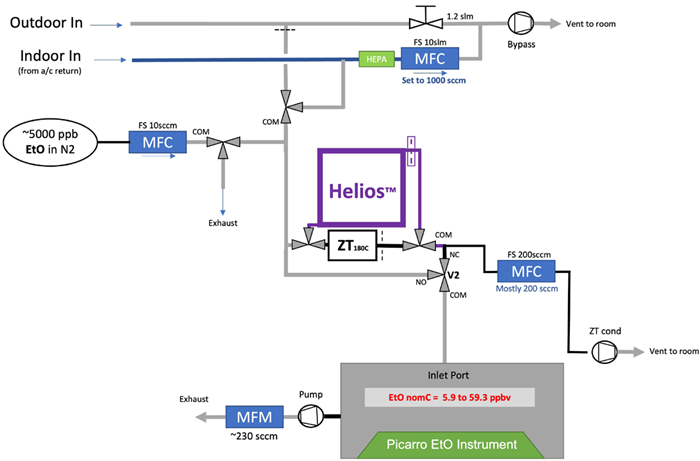
Figure 6. Testing setup for assessing the Helios performance in real-world ambient conditions (slightly simplified for clarity). Image Credit: Picarro
Real-world data shows a range of typical concentrations and air matrices in sterilizer settings. These scenarios were then simulated with the experimental conditions displayed.
In each scenario, EtO was introduced into the Picarro WMS and the Helios (and often a third-party reference material) to determine true zero values and inlet and outlet EtO values (the latter being the EtO concentration exiting the Helios).
Some experiments add additional gases, including acetone, IPA, ambient CO2, CH4, and H2O, to evaluate Helios’ performance and DRE under real-world conditions.
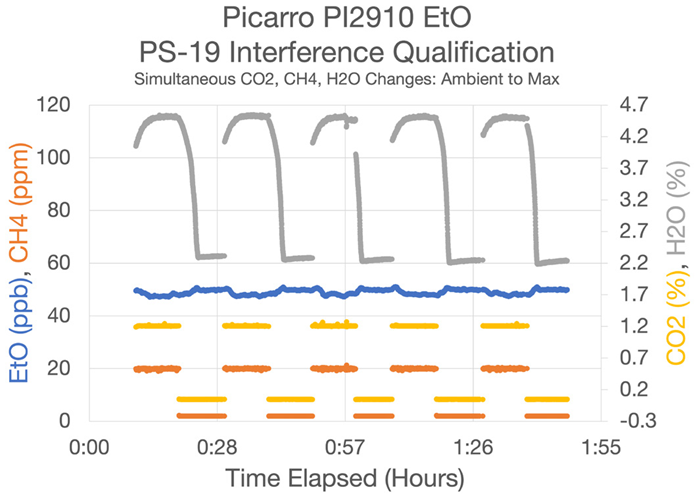
Figure 7. PS-19 Results—Test data produced during EPA Performance Specification 19 analyzer qualification. Here, Picarro chose to introduce all interferent compounds to the gas stream simultaneously, the most challenging scenario to pass. Even with ultra-high CO2 well beyond what is seen in ambient settings, and water vapor equivalent to an Amazonian forest after a rain shower, the most affected instrument showed only a ~1.4 ppb bias. Image Credit: Picarro
Most notably for the experimental setups described, the core Picarro CRDS instrument within the WMS is highly resistant to interferences, possessing the ability to correct for any possible bias arising from a range of compounds, including CO2, CH4, H2O (Figure 7), ammonia, ethylene, and methylene chloride.
It is also highly resilient to bias from other compounds found at commercial sterilizer sites; for example, acetaldehyde, an impurity in an isomer of EtO, is found at an approximately <0.5% level in EtO supplied for sterilization (Figure 8).
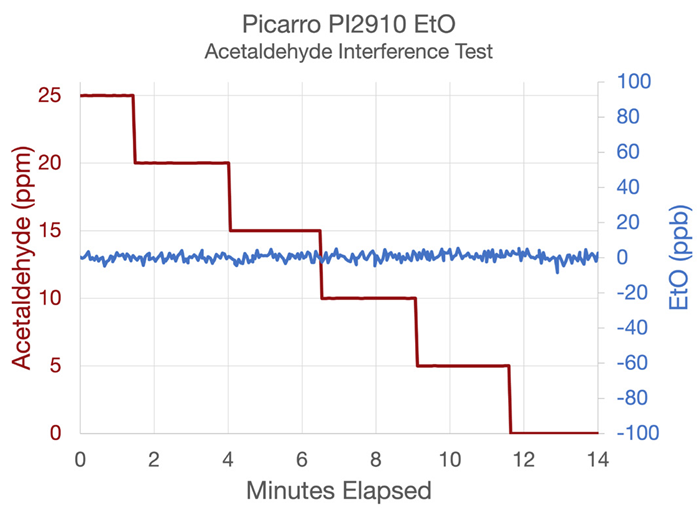
Figure 8. EtO concentrations when challenged with acetaldehyde (note the 250x larger Y axis range for acetaldehyde). Even with a heavily enriched acetaldehyde level of 25 ppm, the EtO showed no bias. Sterilizer GCs typically struggle with this challenge gas, since EtO and acetaldehyde are isomers, and typically co-elute. Image Credit: Picarro
Picarro has also fitted its EtO CRDS instruments with a data quality indicator that searches for deviations in the fit quality and flags all affected EtO data. This acts as a final backstop against very abundant or unknown compounds.
Extensive quality checks were performed during the experiments described. These were done using the gases mentioned to ensure that Picarro’s spectroscopic corrections precisely characterized EtO concentrations despite these other gases' presence.
This was key to confirming that the destruction efficiencies shown were exclusively due to the Helios system’s abatement performance.
In all cases, the system delivered excellent performance, completely unaffected by the major changes in multiple matrix gases. Its performance confirms that it is a highly suitable instrument for the types of testing presented here, as well as highlighting its suitability for handling the types of matrix changes commonly found in sterilizer facilities.
Workplace Setting One: Trapped and Permeating EtO at High Concentrations
Elevated EtO levels in office areas adjacent to a PTE or other process area (Figure 9) are not uncommon in sterilization facilities.
These areas are frequently climate-controlled via a dedicated HVAC system distinct from the plant’s main areas, which provides low humidity and simple air matrices.
Their proximity to high-use areas where a product is sterilized or sits following sterilization means these areas exhibit persistently high EtO levels.
EtO may be introduced in a number of ways in these environments, such as by frequent foot traffic through doorways, particularly when warmer air from process areas rushes into cooler office spaces.
It is also possible for EtO to permeate these areas through cracked or permeable wall materials. This effect can vary depending on how well pressure differentials are maintained between building areas.
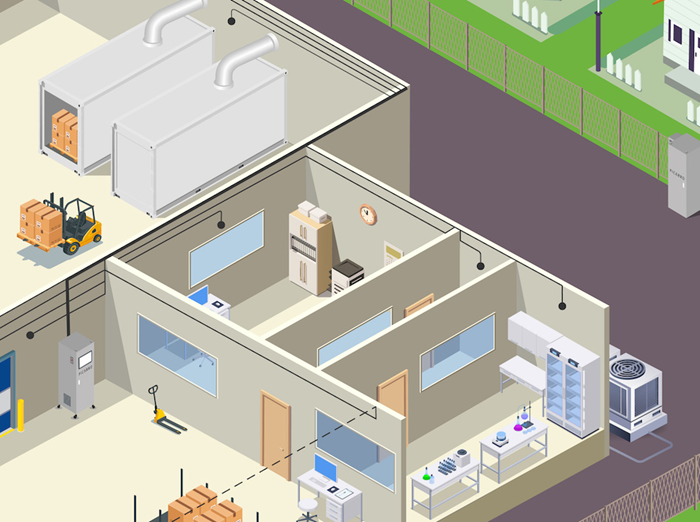
Figure 9. Workplace Setting One: At-risk spaces near process areas. These areas have higher fugitive EtO concentrations than others. Image Credit: Picarro
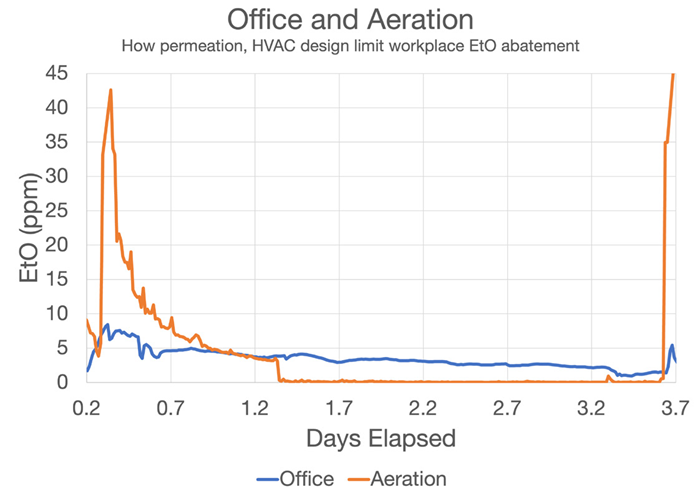
Figure 10. Office (blue) and Aeration (orange) Facility EtO time series data, showing high concentration (ppm levels) in office spaces adjacent to process areas. Proximity to sterilization areas has a clear effect on EtO concentrations in seemingly protected spaces. Image Credit: Picarro
Figure 10 shows data from an aeration room outlet vent and an office adjacent to the aeration chamber. Upon commencement of chamber unloading and aeration room loading (hour 0.2), EtO concentration in the aeration chamber quickly increases to more than 40 ppm.
This change is anticipated from recently sterilized goods, but it is also important to note that EtO concentrations elsewhere in the facility also rapidly increase due to outgassing from these newly unloaded pallets. This is evidenced by the simultaneous uptick in the blue office space tracer.
EtO concentrations in the aeration chamber rapidly drop to approximately 3 ppm following mark 1.2 (around 1 day later), before reducing to less than 1 ppm as the product is removed from the chamber.
Concentration in the office area remains high, however, even two days after the aerated product is removed. These concentrations rise again once pallets are loaded into the aeration chamber a second time. The magnitude of the effects highlighted here is not seen at every facility but is not especially rare.
Figure 9 shows an isometric graphic for the example facility, highlighting how the aeration cells and sterilization chambers on the left have been set in a PTE to manage both process and fugitive emissions.
Even in facilities that do not feature PTEs, areas close to aeration and sterilization tend to be constantly supplied by fresh air from large building fans and periodically from loading docks, which help reduce ambient levels of EtO.
However, the office space shown here is functioning on a dedicated HVAC circuit with no fugitive gas scrubbing. Venting previously cooled air is inefficient, so a significant portion of this air is recirculated.
In this scenario, recirculation traps EtO within these air-conditioned spaces, causing it to remain at single-digit ppm levels for long periods, even after the primary source of EtO has been removed from the adjacent aeration chamber.
The initial set of Helios assessments has been designed to simulate this particular setting. It is expected that abatement in this scenario could be achieved with room-specific Helios units located at the desktop or in-duct units integrated into the air conditioning system.
It was possible to replicate these conditions by sending a simple EtO-in-air gas matrix to the Helios at concentrations that slowly increased from 56 to 5000 ppb (5 ppm). This is approximately the upper limit observed in the office space data shown in Figure 10, using a laboratory setup similar to the one shown in Figure 6.
Table 1 displays the inlet EtO, outlet EtO, and calculated DRE for all EtO levels tested. RH was set to 40% during testing - a value typical of many indoor AC systems where relative humidity (RH) is kept low to discourage mold growth.
The Helios achieved a DRE above 99% in every case, highlighting its impressive removal efficiency across a wide range of EtO concentrations. The results also highlight that up to the current OSHA 8-hr TWA Permissible Exposure Limit (PEL), a single pass is enough to abate EtO to less than the 10 ppb FIFRA PID level suggested by the EPA in its early FIFRA PID announcements.
Figure 11 shows the results of additional DRE testing as a function of RH using an EtO inlet concentration of 1.2 ppm.
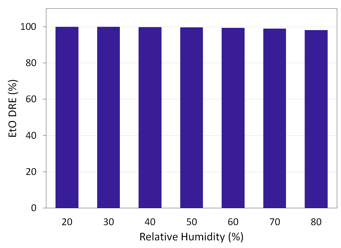
Figure 11. Helios 2.0 EtO DRE as a function of RH. Image Credit: Picarro
In the example presented here, high abatement (99+% DRE) was possible at up to 60% RH. A slight decrease (98+% DRE) was observed at higher RH, but this was found to be negligible.
The Helios demonstrates impressive single-pass EtO DRE, but it should be noted that EtO concentration dynamics in a target area are highly complex. A high DRE EtO control product can continually reduce ambient EtO levels throughout the space as the room air cycles through the reactor.
Further experiments can be performed to characterize Helios products’ in-room performance in sterilization facilities, as well as throughout the downstream medical device supply chain.
Table 1. Helios 2.0 EtO DRE for typical workplace concentrations. Source: Picarro
EtO Inlet (ppb) |
EtO Outlet (ppb) |
DRE (%) |
56 |
ND* |
99.6** |
140 |
0.4 |
99.7 |
330 |
1.7 |
99.5 |
500 |
2.5 |
99.5 |
1000 |
6.4 |
99.4 |
5000 |
25 |
99.5 |
*below detection limit of 0.25 ppb
**assessed using detection limit of 0.25 ppb
Workplace Setting Two: Warehouse Areas near Bay Doors
Low levels of VOCs and other gases are frequently found in warehouse environments. These are typically entrained into the facility through open bay doors or forced air fans (Figure 12).
These settings tend to feature high flows of air piped in from outside. When bay doors are opened, negative pressure gradients from building circulation systems draw in fresh air and exhaust fumes from the bay doors.
Because of this, these areas tend to exhibit lower ambient EtO levels than process areas and potentially lower EtO levels than climate-controlled areas. This is highlighted in Figure 13, most notably at time mark 1, where values dip to almost 0 ppb.
The Helios achieved 98%+ DRE in both indoor and outdoor ambient air matrices, even with inlet values at a low 30.5 ppb.
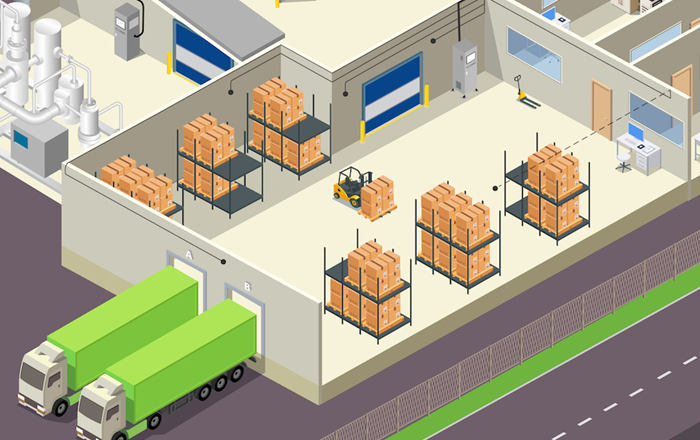
Figure 12. Workplace Setting Two: Warehouse/Quarantine Areas near bay doors where outdoor air is frequently entrained. Image Credit: Picarro
The air in this setting is less conditioned than the first scenario’s climate-controlled air, meaning that Helios’ efficiency in removing EtO in these environments depends on its ability to function in humid and extremely complex gas backgrounds.
A series of tests was performed using standard addition methods to assess the EtO DRE of the Helios in real-world ambient outdoor and indoor air matrices.
The laboratory testing setup displayed in Figure 6 was configured during these tests to introduce outdoor or indoor air at predictable ratios. This included EtO gas to provide a low concentration of 30.5 ppb EtO, a commonly encountered concentration close to bay doors in sterilizer facilities.
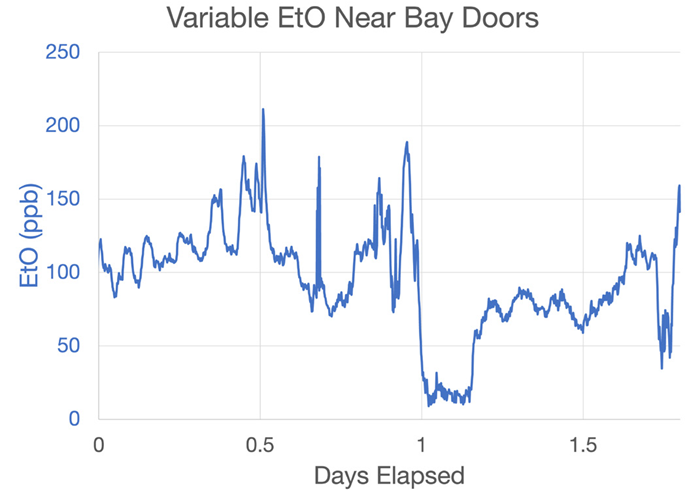
Figure 13. Data from an area of the warehouse adjacent to the shipping bay doors showing frequent dips and rises associated with opening and closing of these bays and other facility doors. Image Credit: Picarro
Table 2 shows the average results of these experiments following several iterations. The resulting DRE for outdoor settings was determined to be 98.4%, while the DRE for indoor air was determined to be 99.1%.
These two efficiencies can be considered almost equivalent at these low levels where the interpretation is limited by the method detection limit of the experiment (0.25 ppb in this case). However, there is a small chance that outdoor air is more challenging to scrub effectively than indoor air.
Even at these low levels, the room-temperature removal of EtO at 98+% DRE highlights the effectiveness of the Helios control technology at removing EtO. This is also the case in air matrices complicated by wide-ranging RH and other contaminants.
Table 2. Helios 2.0 EtO DRE of standard-added outdoor and indoor air. Source: Picarro
Interferent |
EtO In (ppb) |
EtO Out (ppb) |
DRE (%) |
Outdoor Air |
30.5 |
0.5 |
98.4 |
Indoor Air |
30.5 |
ND* |
99.1** |
*below detection limit of 0.25 ppb
**assessed using detection limit of 0.25 ppb
Workplace Setting Three: Complex Air Matrices, Laboratory Settings
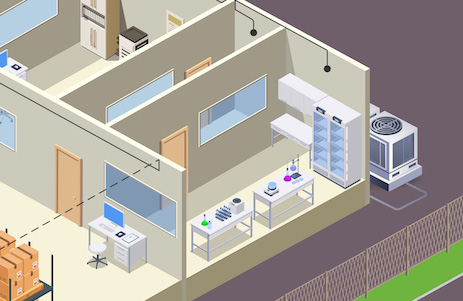
Figure 14. Workplace Setting Three: Areas where other common chemical are used, e.g., laboratory settings, kitchens, janitorial spaces. Image Credit: Picarro
EtO electrochemical sensors often respond when solvents or cleaning agents are used nearby. Due to this uncertainty around false positives, facilities may struggle to assess their risk in settings like laboratories where other chemicals may be stored and used (Figure 14).
This is an important consideration because many workplace settings feature the presence of VOCs and other gases; for example, cleaning agents such as acetone and IPA. They also regularly include perfumes and deodorants worn by workers.
Picarro systems can deliver reliable data in the face of complex matrices, thanks to the company’s extensive work in factory settings.
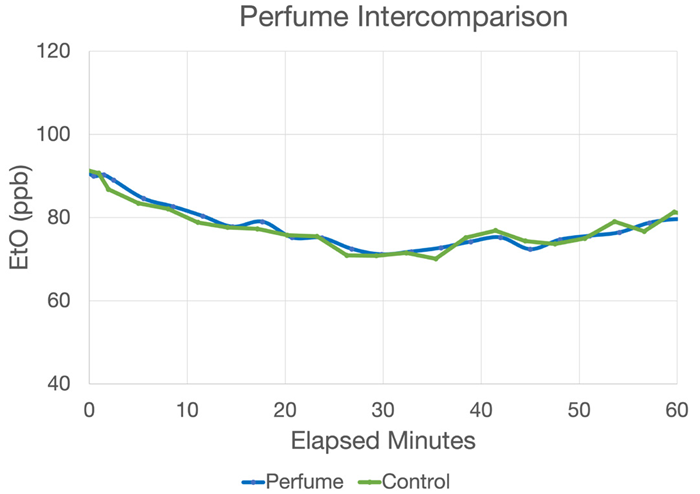
Figure 15. Picarro data at a commercial sterilizer showing that heavy, immediately adjacent perfume loads had no effect on the ambient Picarro readings (~80 ppb) compared to the control. Image Credit: Picarro
Figure 15 shows seven fresh perfume tabs featuring various alcohols and fragrances set up 1 inch away from the Workplace Monitoring System’s inlet (blue). An identical line (green) was also stretched out 6 feet to the side to function as a control dataset.
Data was acquired over a period of around one hour to compare the two values reported. The two datasets show near-perfect agreement over that time. Combined with other demonstrations of resilience to interferents, this data provides robust evidence of the Picarro WMS’s performance in complex matrices.
In the lab setting with the Helios 2.0, ambient air was combined with carefully regulated levels of either IPA or acetone, along with a consistent regulated amount of EtO to assess the impact of these VOCs on EtO DRE.
Table 3 shows EtO inlet and outlet concentrations and DRE at two inlet concentrations. The top half of the Table features data with EtO inlet values at 990 ppb (~1 ppm), while the bottom half features data with EtO inlet values at 1460 ppb (~1.5 ppm). This comparison is to determine whether the concentration of EtO affects DRE.
The Helios was initially permitted to only abate EtO coming from the tank before the interferent (IPA or acetone) was introduced. This was the case in both experiments.
Next, the interferent was added at values similar to those found in workplace settings, near 8-hr PELs to demonstrate its effect on the EtO DRE.
In every scenario evaluated, the Helios delivered 99.9+% DRE in a pure EtO stream, with only minimally reduced efficiency when the interferent gases were introduced (typically 99.8%).
These results highlight the Helios system’s ability to remove EtO, even in the presence of common VOCs.
Table 3. Helios DRE with relevant ambient concentrations of acetone and IPA interferents. Source: Picarro
Interferent |
EtO In (ppb) |
Interferent (ppm) |
EtO Out (ppb) |
EtO DRE (%) |
Acetone |
990 |
0 |
0.36 |
99.9+ |
Acetone |
990 |
1 |
1.07 |
99.9 |
Acetone |
990 |
3.1 |
2.18 |
99.8 |
IPA |
990 |
0 |
0.34 |
99.9+ |
IPA |
990 |
13 |
1.04 |
99.9 |
IPA |
990 |
63 |
2.32 |
99.8 |
Acetone |
1460 |
0 |
0.92 |
99.9 |
Acetone |
1460 |
2.8 |
2.06 |
99.8 |
IPA |
1460 |
0 |
0.93 |
99.9 |
IPA |
1460 |
56 |
3.62 |
99.8 |
Conclusions
This article featured data acquired via Picarro Workplace Monitoring Systems in three different workplace settings in participating commercial sterilizers.
Data from this workplace monitoring systems highlighted the core CRDS technology’s excellent resiliency to interferences and carryover and showcases its capacity to represent concentrations across a wide dynamic range.
Critical situational information was provided around the real-world conditions within EtO sterilizers, allowing experiments to be developed to thoroughly evaluate the real-world performance of Sonata Scientific’s Helios abatement systems.
The experiments presented here confirm that the Sonata Scientific Helios systems can easily accommodate inlet conditions ranging from (but not limited to) 30.5 to 5000 ppb, consistently achieving excellent DRE above 98.4% for low concentrations and as high as 99.9% in most circumstances.
It was also demonstrated that these efficiencies remained in place despite challenging air matrix elements like acetone, isopropyl alcohol, CO2, CH4, and H2O. These results confidently indicate that the Helios system is ideally suited for laboratory, office, and warehouse settings.
The 100 CFM EtO control system from Sonata Scientific is currently being assessed at several select medical device sterilization facilities. These evaluations have achieved DRE above 99%, consistent with the results reported in this article.
Sonata is also in the process of developing a 500 CFM product, and the company continues to target higher CFM flow rates commonly associated with commercial ducting and blower fans.
References and Further Reading
- McDonald Process, US Patent No. 3,068,064A
- Revised NESHAP: https://www.federalregister.gov/documents/2023/04/13/2023-06676/national-emission-standards-for-hazardous-air-pollutants-ethylene-oxide-emissions-standards-for
- FIFRA PID: https://www.federalregister.gov/documents/2023/04/13/2023-07727/pesticide-registration-review-proposed-interim- decision-and-draft-risk-assessment-addendum-for
Acknowledgments
Produced from materials originally authored by Jonathan Bent, Ph.D. from Picarro and Melissa Petruska, Ph.D. from Sonata Scientific.

This information has been sourced, reviewed and adapted from materials provided by Picarro.
For more information on this source, please visit Picarro.