Introduction The nitrogen oxides NOx are undesired air pollutants since they are highly toxic and are one of the main reasons for the appearance of acid rain and smog. Therefore the NOx removal from different waste gases is a central problem in modern industry. One of the most widely applied techniques for deNOx is selective catalytic reduction (SCR). A number of reports [1-8] have shown that carbon monoxide, ammonia and hydrocarbons can be used as reductants. The selective catalytic reduction of NOx with hydrocarbons was studied over a variety of catalysts, that are metal ion- exchanged zeolites such as Cu/ZSM-5 [9, 10], Co/ZSM-5, Co/FER, Fe/ZSM-5, Pt/ZSM-5, Co-Pt/ZSM-5 [11,12], metal and metal oxide- supported catalysts [13-16], perovskite- type oxides (like LaAlO3, SrSnO3, CaTiO3) [17], spinel- type oxides (like NiGa2O4, ACr2O4 (A= Cu, Mg, Co, Mn), CoMn2O4, AFe2O4 ( A= Cu, Co, Ni) ) [18, 19]. The majority of the catalysts were prepared by wet ion exchange, wet impregnation, co-precipitation and sol-gel methods. Numerous mixed oxide-catalysts could be synthesized at much lower temperatures by sol-gel method than by the conventional ceramic method, they also had higher purity than by co-precipitation method and high activity. In this study, we prepared CoAl2O4 and NiAl2O4 spinels by citrate gel method. The prepared samples were characterized by X- ray diffraction (XRD), temperature-programmed desorption (TPD), temperature-programmed reduction (TPR) and nitrogen adsorption and evaluated for NOx reduction with n- hexane. Experimental Catalyst Preparation Citric acid was added to the mixed aqueous solution of metal nitrates in the desired proportion so that the molar ratio of citric acid to the metal to be 0.6, 0.7 and 0.8. The mixed aqueous solutions were evaporated with continuously stirring, while simultaneously adding aqueous ammonia for adjusting the final suitable pH until the solution was converted to gel. The gel was dried at 80oC overnight, transferred to xerogel, followed by calcination at 550, 700, 800oC for 4 h for CoAl system and 550, 700, 800, 850 and 900oC for 4 h for NiAl system. Catalyst Characterization The X-ray diffractograms of these samples were recorded on a diffractometer (Siemens model D- 5005) with a Cu Kα radiation, scanning from 15 to 70o using a scan step of 0.03o with a step time of 1.0 s. H2 reduction experiments were performed by a TPR apparatus (Autochem II 2920, USA), equipped with a TCD. A sample (0.07-0.09 g) was pretreated in a flow of 5%O2/He at 500oC for 1 h and then cooled to room temperature. Lastly the reduction of the sample was carried out in an H2/Ar (10.4%) flow of 40ml/min from room temperature up to 1000oC with a ramp of 10oC/min. The same TPR apparatus was used for CO-TPD experiments. Each experiment was started with a pre-treatment of the catalyst sample of 0.08-0.1 g in 10.2%O2/He at 500oC for 2 h. Subsequently, the sample was cooled down to room temperature, at which a gas flow with 10.2%CO in He was passed over the catalyst until saturation of the catalyst surface was reached. Then the sample was purged in He flow for 60 min to remove all physisorbed species. Finally, TPD was carried out in pure He at a heating rate of 5oC/min. The BET surface area, pore size distribution and pore volume of the prepared catalysts were determined from N2 isotherms measured at -196oC by using a micropore analyzer (ASAP 2010, USA). NOx Reduction with n- hexane Catalytic activity was tested in a continuos flow reactor system consisting of a vertical quartz tube reactor (3mm inner diameter), the catalyst of 0.05 g fixed by quartz wool. Prior to the reaction, the sample was pretreated with flowing pure O2 at 500oC for 1 h, cooling down to room temperature and finally purging in N2 flow. NOx reduction was carried out by feeding a gas mixture containing 1000 ppm NO, 50 ppm NO2, 6000 ppm n- hexane, 5% O2 and N2 as a carrier gas. n-hexane was introduced by passing part of the carrier gas to the saturator. The total gas flow rate was 70 ml/min, corresponding to the space velocity GHSV of 84,925 h-1. NOx concentration of inlet and outlet gas mixture was measured by a Quintox sensor (UK). Results and Discussion The Formation of the Prepared Spinels Figures 1 and 2 show the XRD diagrams of xerogel samples of CoAl system with various molar ratio of citric acid to the metals and calcination temperatures. From the XRD diagram of xerogel with the molar ratio of citric acid to the metals of 0.6, calcined at 550oC shown in Figure 1a, it is clear that all the diffraction peaks of CoAl2O4 spinel were present, nevertheless some weak diffraction peaks corresponding to a variety of Al2O3 and a few weak peaks of CoO were also observed. By increasing the temperature up to 700oC the XRD pattern in Figure 1b shows that only several diffraction peaks of Al2O3 were found and at 800oC (Figure 2c) the system became a single phase- CoAl2O4 spinel. Then as a result of the enhancement of the molar ratio of citric acid to the metals up to 0.7 (Figure 2d) a single phase- CoAl2O4 was obtained at lower temperature of700oC. This phase was even crystallized more than the above CoAl2O4, formed when xerogel with the molar ratio of 0.6 was calcined at 800oC. Obviously these experiments prove citric acid to be an important agent for making gel and a bridge for linking metal cations to form a citrate complex. Therefore diffusive distance of metal cations in solid-state reaction was decreased and resulted in relatively decreasing calcination temperature needed for spinel formation. (a) Xerogel of CoAl system (0.6-550°C) 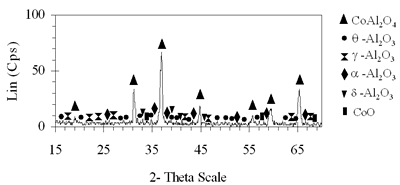 (b) Xerogel of CoAl system (0.6-700°C) 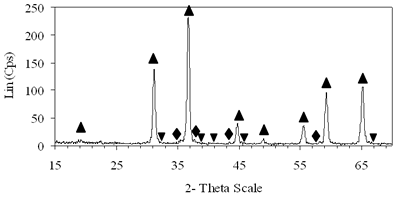 | Figure 1. XRD patterns of xerogels of CoAl system, calcined for 4 h at (a) 550°C, (b) 700°C with the molar ratio of 0.6. | (c) Xerogel of CoAl system (0.6-800°C) 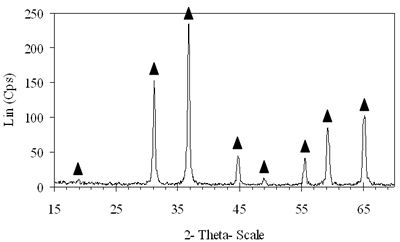 (d) Xerogel of CoAl system (0.7-700°C) 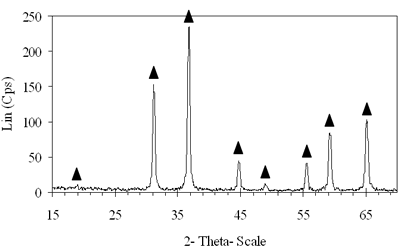 | Figure 2. XRD patterns of xerogels of CoAl system, calcined for 4 h at (c) 800°C with the molar ratio of 0.6 and (d) 700°C with the molar ratio of 0.7. | The formation of NiAl2O4 spinel with increasing calcination temperature and the acid:metal molar ratio is also given as illustrated in Figures 3 and 4. The XRD diagram of xerogel with the molar ratio of 0.6, calcined at 550°C (Figure 3a) shows the initial formation of NiAl2O4 spinel as can be seen the appearance of a few weak diffraction peaks of NiAl2O4. In general at this condition the system mainly consists of two oxides: NiO, Al2O3. As the temperature increases up to 700°C shown in Figure 3b the crystalline lattice was not developed considerably. However, with an increase in the molar ratio of citric acid to the metals and calcination temperature, the XRD pattern of xerogel with the molar ratio of 0.7 and calcination temperature of 800°C (Figure 4c) shows that the system was better crystallized, but the weak diffraction peaks of NiO were also present. With calcination temperature of 850°C (Figure 4d) the system was almost unchanged, so the acid:metal molar ratio and calcination temperature were continually increased for increasing diffusive rate of the metal cations in solid-state reaction. The xerogel with the molar ratio of 0.8, calcined at 900°C as observed in Figure 4e formed the spinel, which was much better crystallized, the diffraction peaks of NiAl2O4 became very sharp, but the trace of NiO remained in the phase. (a) Xerogel of NiAl system (0.6-550°C) 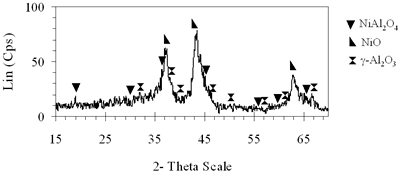 (b) Xerogel of NiAl system (0.6-700°C) 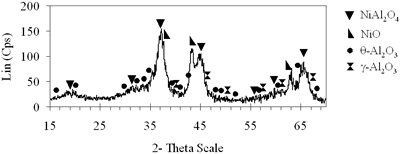 | Figure 3. XRD patterns of xerogels of NiAl system, calcined for 4 h at (a) 550°C, (b) 700°C with the molar ratio of 0.6. | (c) Xerogel of NiAl system (0.7-800°C) 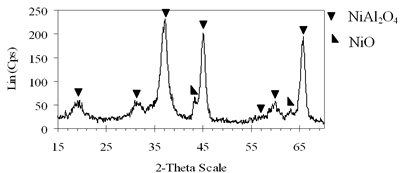 | (d) Xerogel of NiAl system (0.7-850°C) 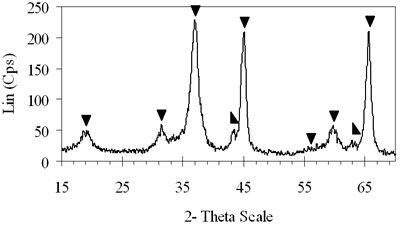 | (e) Xerogel of NiAl system (0.8-900°C) 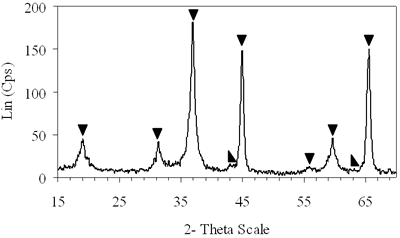 | Figure 4. XRD patterns of xerogels of NiAl system, calcined for 4 h at (c) 800°C, (d) 850°C with the molar ratio of 0.7 and (e) 900°C with the molar ratio of 0.8. | H2-TPR of the Prepared Catalysts H2-TPR profile of 0.8-900°C NiAl2O4 spinel presents in Figure 5a, there is a small and broad reduction peak with maximum at 470°C and another one with strong intensity centered at 790°C. Experimentation indicated that pure NiO could be reduced at about 400°C [21]. Therefore the small peak at 470°C in the TPR profile of NiAl spinel represents the reduction of a small amount of NiO, which aggregates on the catalyst surface as an impurity. The main reason for the peak at a higher temperature lies in the interaction between the aggregated NiO and the surface of the crystal lattice [21]. The second-high temperature peak can be assigned to the reduction of Ni2+ ions in the lattice of the spinel. It is revealed that the Ni2+ ions incorporated in the crystalline lattice were more difficult to be reduced than NiO [20]. The hydrogen consumption during the reduction of NiAl2O4 spinel also displays in Table 1. Based on the data listed below, we calculated that approximately 97.6% of Ni2+ ions in the lattice could be reduced to Ni0 at 790°C. Figure 5b shows that on 0.7- 700°C CoAl2O4 spinel there is one main peak centered at 870°C. This peak should be attributed to the reduction of Co2+ ions in the lattice, except for a small peak at 540°C due to reduction of an impurity. This explanation completely agrees with those of Arnoldy et al. [22]. However, the impurity seems to be a small amount of CoO or Co3O4, located on the surface of CoAl2O4 spinel that was almost invisible by XRD. Arnoldy et al. also showed that single CoO and Co3O4 could be reduced at 337°C and 317°C respectively [22]. That means the cobalt oxide on the surface of the prepared spinel was more difficult to be reduced than single oxide because of the interactions between the cobalt oxide and the surface of the spinel. From the data in Table 1 it was found that all the Co2+ ions in the lattice could be reduced to Coo at 870°C. 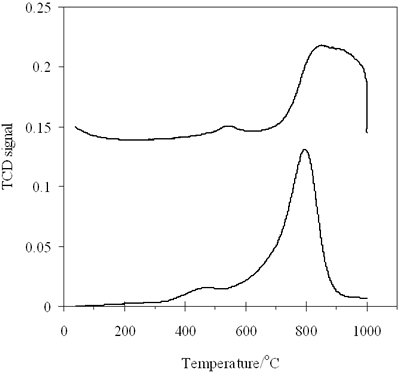 | Figure 5. H2- TPR profiles of (a) 0.8-900oC NiAl2O4 and (b) 0.7-700 CoAl2O4 spinels. | Table 1. Hydrogen consumption in TPR of 0.8-900 NiAl2O4 and 0.7-700 CoAl2O4 | | Peak1 | Peak 2 | Peak 1 | Peak 2 | Peak 1 | Peak2 | | NiAl2O4 | 470 | 790 | 10.396 | 123.725 | 0.464 | 5.5234 | 5.9875 | CoAl2O4 | 540 | 870 | 11.024 | 126.789 | 0.492 | 5.665 | 6.157 | Hence it is concluded that TPR is a sensitive method to characterize surface properties of a catalyst. Reducibility, interaction between phases and also purity of catalyst can be evaluated by TPR method. As the above TPR data it is observed that 0.8-900oC NiAl2O4, as well as 0.7-700oC CoAl2O4 is well crystallized. The majority of Ni2+ and Co2+ ions come into the spinel lattice, nevertheless there is a small amount of these ions existing in oxide type, located on the surface of the spinels as an impurity. The TPR results are in agreement with the above XRD results, confirming high crystallinity of the prepared spinels, moreover TPR method appears more sensitive than XRD due to the detection of trace components. CO- TPD of the Prepared Catalysts Figure 6 shows CO-TPD profiles of both catalysts, which are similar. The TPD pattern of NiAl spinel (Figure 6a) characterized by two desorption peaks, one with strong intensity at 89oC and the other -small peak located at 640oC. It means that, CO adsorption over 0.8-900oC NiAl sample occurs on two adsorptive site groups. The first group gives the strong peak ranging from 70 to 300oC, which species over the catalyst. The second one is characteristic of the strong chemically adsorbed CO molecules. 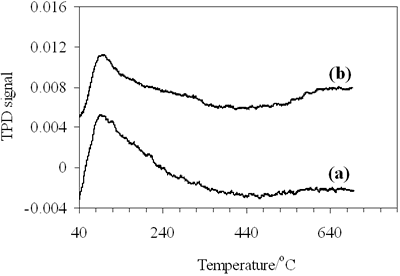 | Figure 6. CO- TPD profiles of (a) 0.8-900oC NiAl2O4 and (b) 0.7-700oC CoAl2O4 spinels. | The TPD profile of CoAl spinel (Figure 6b) consists of three desorption peaks, the first one with strong intensity at 96oC and two peaks with medium intensity as well, one at about 310oC and the other at 660oC, but the desorption peak at 310oC is not well resolved with the low temperature peak at 96oC. Thus 0.7- 700oC CoAl2O4 showed three adsorptive site groups, the first one attributed to the weak adsorptive site group, the second-the medium and the third -the strong. The data in Table 2 show that the amount of chemisorbed CO on NiAl2O4 spinel is much more than on CoAl2O4 spinel, specially on the weak adsorptive sites, corresponding to the low desorption temperatures at 89oC and 96oC, respectively the amount of adsorbed CO on NiAl2O4 spinel is three times as much as on the other. The difference may be related to different electron configurations of nickel and cobalt in the spinels or different geometry states of the adsorptive sites on NiAl and CoAl spinels. Table 2. Chemisorption of Carbon Monoxide on 0.8-900oC NiAl2O4 and 0.7-700oC CoAl2O4. | | Peak1 | Peak 2 | Peak 3 | Peak 1 | Peak 2 | Peak 3 | | NiAl2O4 | 89oC | - | 640oC | 1.745 | - | 0.234 | 0.088 | CoAl2O4 | 96oC | 310oC | 660oC | 0.540 | 0.244 | 0.346 | 0.050 | In order to investigate surface state of these spinels we measured its nitrogen adsorption isotherms. The data and calculated results from the isotherms are illustrated in Figure 7 and listed in Table 3. 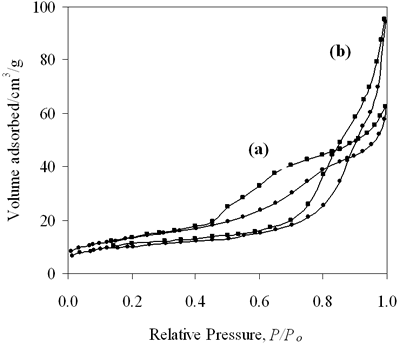 | Figure 7. N2 adsorption isotherms for (a) 0.8-900oC NiAl2O4 and (b) 0.7-700oC CoAl2O4 spinels. | Table 3. Characteristics of 0.8-900oC NiAl2O4 and 0.7-700oC CoAl2O4 spinels. | 0.8-900 NiAl | 48 | 6.1 | 0.099 | 0.7-700 CoAl | 38 | 14.8 | 0.147 | Table 3 also summarizes the BET surface area, the pore size and the pore volume of these samples. It is seen that the BET surface area of NiAl spinel is larger than that of CoAl spinel, the pore size and the pore volume of the first are narrower than that of the second. The N2 adsorption isotherms display that both catalysts present hysteresis loops, so the catalysts are mesoporous. From the results we found that the BET surface area of NiAl spinel is slightly larger than that of CoAl spinel, however the amount of chemisorption CO on NiAl spinel at the low temperature is three times as much as on the other. This indicates that the difference in adsorptive properties between these spinels must be related to different electron configurations of nickel and cobalt in the crystalline lattice. Selective Reduction of NOx with n- Hexane as a Reductant Figure 8a shows the results for the selective reduction of NOx over 0.8-900oC NiAl2O4 spinel, the reaction temperature varying from 100 to 350oC. The data on the curve indicate that the catalyst appears very high active at low temperatures, at a temperature as low as 100oC the conversion of NOx was approximately 94%. 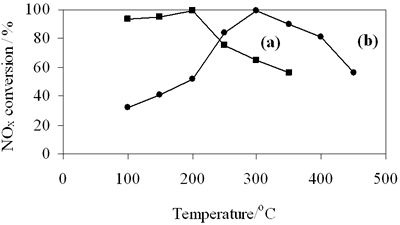 | Figure 8. NOx conversion on (a) 0.8-900oC NiAl2O4 and (b) 0.7-700oC spinels. | The selective reduction of NOx over 0.7-700oC CoAl2O4 was carried out with the reaction temperature between 100-450oC. Figure 8b shows NOx conversion as a function of the reaction temperature. By increasing the reaction temperature NOx conversion increases to reach a maximum value of 99% at about 300oC and above 350oC it drastically decreases. It is also seen in Figure 8 that the selective catalytic reduction of NOx with n-hexane (n-hexane-SCR) mainly occurs in the low temperature range of 100-350oC. So the weak adsorptive sites can be catalytic active sites for n-hexane-SCR over these spinels, because in the low temperatures the number of the weak adsorptive sites on NiAl spinel is three times as much as on CoAl spinel, at the same time NOx conversion in the range of 100- 200oC over NiAl spinel is twice or three times (see Figures 8a and 8b). Above 200oC NOx conversion on NiAl spinel decreases, but continually increases on CoAl spinel, which is probably due to existence of the medium adsorptive sites (at 310oC) on CoAl spinel. As far as we know based on mechanism of HC-SCR the selective reduction of NOx is initiated by adsorption of hydrocarbon and/or NO, forming intermediate complex over catalyst surface [17]. So in this case catalytic activity for SCR of NOx closely relating to adsorptive characteristic of catalyst is entirely possible. In addition Figure 8a shows that NiAl spinel has the active temperature window in the low temperature region ranging from 100 up to 200oC. It is particularly advantageous to prepare NiAl spinel, compared to another catalysts for SCR of NOx such as metal ion-exchanged zeolites, Pt/Al2O3, etc. It is also technology aim on which researchers are focusing to solve NOx elimination from the exhaust of diesel engines as well as industrial waste sources. The above results of catalytic properties are preliminary, but attracting considerable interest, especially NiAl2O4 spinel seems to be a promising and potential catalyst due to its low active temperature window. Conclusion CoAl2O4 and NiAl2O4 spinels were successfully prepared at comparatively low temperatures by citrate gel method. The XRD and TPR results confirm that the prepared spinels have high crystallinity and trace impurities. n-Hexane- SCR of NOx performs at the low temperatures over the prepared spinels. The chemisorption and activity test results correspond to NiAl spinel at high NOx conversion at lower temperatures than CoAl spinel to having a larger number of the weak adsorptive sites compared to the other. However, BET surface area of the first does not strongly differ from that of the latter. So the difference in adsorptive properties between two spinels may be due to different electron configurations of nickel and cobalt in these spinels. Acknowledgements The authors are grateful to Professor Nguyen Huu Phu from Department of Catalysis and Surface, Institute of Chemistry, National Center for Science and Technology of Viet nam for experiment support and valuable discussions. References 1. J. W. London and A. T. Bell, “A Simultaneous Infrared and Kinetic Study of the Reduction of Nitric Oxide by Carbon Monoxide over Copper Oxide”, J. Catal., 31 (1973) 96-109. 2. T. Chafik, D. I. Kondarides and X. E. Verykios, “Catalytic Reduction of NO by CO over Rhodium Catalysts”, J. Catal., 190 (2000) 446- 459. 3. H. Niiyama, K. Murata and E. Echigoya, “The Mechanism of the Reaction of Nitrogen Oxide with Ammonia over Cr2O3-Al2O3 and Cr2O3 Catalysts”, J. Catal., 48 (1977) 201-208. 4. S. Bröer and T. Hammer, “Selective Catalytic Reduction of Nitrogen Oxides by Combining a Non-Thermal Plasma and a V2O5-WO3/TiO2 Catalyst”, Appl. Catal. B: Envir., 28 (2000) 101-111. 5. F. C. Meunier, J. P. Breen, V. Zuzaniuk, M. Olsson and J. R. H. Ross, “Mechanistic Aspects of the Selective Reduction of NO by Propene over Aluminum and Silver-Alumina catalysts”, J. Catal., 187 (1999) 493-505. 6. J. Blanco, P. Avila, S. Suarez, J. A. Martin and C. Knapp, “Alumina and Titania Based Monolithic Catalysts for Low Temperature Selective Catalytic Reduction of Nitrogen Oxides”, Appl. Catal. B: Envir., 28 (2000) 235-244. 7. X. Wang, H.Y. Chen and W. MH. Sachtler, “Catalytic Reduction of NOx by Hydrocarbons over Co/ZSM-5 Catalysts Prepared with Different Methods”, Appl. Catal. B: Envir., 26 (2000) L227- L239. 8. K. Hadjiivanov, V. Avreyska, G. Tzvetkov, P. Stefanov, C. Chupin, C. Mirodatos and Ts. Marinova, “Selective Catalytic Reduction of NOx by Methane over Co/ZrO2 Catalysts”, Surf. Interf. Anal., 32 (2001) 175-178. 9. K. Hadjiivanov, D. Klissurski, G. Ramis and G. Busca, “Fourier Transform IR Study of NOx Adsorption on a CuZSM-5 DeNOx Catalyst”, Appl. Catal. B: Envir., 7 (1996) 252. 10. M. Guyon, V. L. Chanu, P. Gilot, H. Kessler and G. Prado, “Experimental Study of the Formation and the Reaction of an Intermediate during the Lean NOx Reaction over CuZSM-5”, Appl. Catal. B: Envir., 8 (1996) 183- 196. 11. A. V. Salker and W. Weisweiler, “Catalytic Behaviour of Metal Based ZSM-5 Catalyst for NOx Reduction with NH3 in Dry and Humid Conditons”, Appl. Catal. A: Gen., 203 (2000) 221. 12. N. W. Cant and I. O. Y. Liu, “The Mechanism of the Selective reduction of Nitrogen Oxides by Hydrocarbons on Zeolite Catalysts”, Catal. Today, 63 (2000) 133- 146. 13. K. I. Shimizu, A. Satsuma and T. Hattori, “Catalytic Performance of Ag-Al2O3 Catalysts for the Selective Catalytic Reduction of NO by Higher Hydrocarbons”, Appl. Catal. B: Envir., 25 (2000) 239-247. 14. E. Seker and E. Gulari, “Activity and N2 Selectivity of Sol-gel Prepared Pt/Alumina Catalysts for Selective NOx Reduction”, J. Catal., 194 (2000), 3-13. 15. T. Maunula, J.Ahola and H.Hamada, “Reaction Mechanism and Kinetics of NOx by Propene on CoOx/Alumina Catalysts in Lean Conditions”, Appl. Catal. B Environ., 26 (2000) 173- 192. 16. L. Lietti, I. Nova, G. Ramis, L. D. Acqua, G.Busca, E. Giamello, P. Forzatti and F. Bregani, “Characterization and Reactivity of V2O5-MoO3/TiO2 De-NOx SCR Catalysts”, J. Catal., 187 (1999) 419- 534. 17. A. Fritz and V. Pitchon, “The Current State of Research on Automotive Lean NOx Catalyst”, Appl. Catal. B: Envir., 13 (1997)1-25. 18. L. Chen, T. Horiuchi and T. Mori, “Catalytic Reduction of NO over a Mechanism Mixture of NiGa2O4 spinel with Maganese Oxide: Influence of Catalyst Preparation Method”, Appl. Catal. A Gen., 209 (2001) 97- 105. 19. W. F., Shangguan, Y. Teraoka and S. Kagawa, “Simultaneous Catalytic Removal of NOx and Diesel Soot Particulates over Ternary AB2O4 Spinel-Type Oxides”, Appl Catal.B: Environ., 8 (1996) 217-227. 20. W. Shan, M. Luo, P. Ying, W. Shen and C. Li, “Reduction Property and Catalytic Activity of Ce1-xNixO2 Mixed Oxide Catalysts for CH4 Oxidation”, Appl. Catal. A: Gen., 246 (2003) 1-9. 21. X.Zhang, J. liu, Y. Jing and Y. Xie, “Support Effects on the Catalytic Behaviour of NiO/Al2O3 for Oxidative Dehydrogenation of Ethane to Ethylene”, Appl. Catal. A: Gen., 240 (2003) 143- 150. 22. P. Arnoldy and J.A. Moulijn, “Temperature Programmed Reduction of CoO/Al2O3 Catalysis”, J. Catal., 93 (1985) 38-51. Contact Details |