Introduction Studies on the use of rare-earth complexes as the emitter materials in OLEDs (organic light emitting devices) have attracted considerable attention recently [1-8]. Unlike common fluorescent and phosphorescent compounds, rare-earth complexes show high luminance efficiency with sharp emission bands involving electrons associated with inner f orbitals of the central rare-earth metal ions. Of the rare-earth complexes reported, europium complexes appear most studied due to the strongly red-emission ability that are widely exploited in full-color displays [2]. Several europium complexes have been employed as red emitters in electroluminescent devices [1-8]. Due to the unique mechanism of excitation, europium complexes used in electroluminescent devices emit red light at the same frequency, although the ligands on the europium center are different to each other [9]. In the fabrication of electroluminescent device using europium complexes as dopants, the choice of host materials and hole blocker is very limited because of the very low-lying HOMO levels for most europium complexes. Several groups have successfully produced saturated red light by using CBP (4,4’-N,N’-dicarbazole-biphenyl) as the host and BCP (2,9-dimethyl-4, 7-diphenyl-1, 10-phenanthroline), TAZ ( 3 - [biphenyl-4-yl]- 4- phenyl- 5- [4-tert-butyl] phenyl-1,2,4-triazole) or the europium complex itself as the hole blocker [1,2,4-6]. In a recent paper [10] reported, we used europium complex Eu(TTA)3(DPPz), where TTA = 2-thenoyltrifluoroacetonate and DPPz = dipyrido[3,2-a:2’,3’-c]phenazine, as the red emitters for electroluminescent devices. Some of these devices emit saturated red light with brightness greater than 1000 cd/m2. In this paper, we prepared phenanthroline derivatives with a strong electron donating amino group and new europium complexes based on these new ligands and the use of these complexes as red emitters in electroluminescent devices. Experimental General Information All reactions were carried out under nitrogen atmosphere in sealed reaction vessels. 1,10-Phenanthroline-5,6-epoxide was synthesized according to a literature method [11]. Piperidine, diethylamine and other chemicals were used as purchased. Melting points were measured on a Fargo MP-2D melting point apparatus and were uncorrected. 1H NMR spectra were recorded with a Varian Mercury 400 spectrometer. High resolution mass spectra were obtained on a Finnigan MAT-95XL instrument. Elemental analysis was carried out using a Heraeus CHN-O Rapid instrument. UV-vis spectra were recorded on a Hitachi U-3300 model while photoluminescence (PL) spectra were taken using a Hitachi F-4500 fluorescence spectrophotometer. OLED Fabrication and Measurement EL devices based on europium complexes were fabricated through vacuum deposition of the materials onto clean glass precoated with a layer of indium tin oxide (sheet resistance 25 ohm/square) at 10-6 Torr. The rate of deposition of each organic compound was 0.1–0.3 nm/s and for the europium complex was 0.005–0.01 nm/s. The cathode was formed through co-evaporation of Mg and Ag with Mg:Ag ratio 10:1 and total thickness 55 nm, followed by vacuum deposition of Ag (100 nm). Rates of co-deposition of Mg and Ag were 0.5–0.6 and 0.05–0.06 nm/s, respectively; the rate of deposition of Ag was 0.3 nm/s. The emitting diode has an effective area 9.00 mm2. Current, voltage and light intensity were measured simultaneously using a source meter (Keithley 2400) and an optical meter (Newport 1835-C) equipped with a silicon photodiode (Newport 818-ST). EL spectra were measured on a fluorescence spectrophotometer (Hitachi F-4500). The Commission Internationale de l’Éclairage (CIE) values were calculated based on the data of EL spectra [12]. According to the CIE 1931 system, X = ΣP(λ)x(λ)dλ, Y = ΣP(λ)y(λ)dλ and Z = ΣP(λ)z(λ)dλ; where P(λ) was the emission intensity of the EL spectrum, x(λ), y(λ) and z(λ) were the color-matching function. Therefore, the CIE values of x and y of a device were equal to X/(X + Y + Z) and Y/(X + Y + Z), separately. Oxidation potentials were obtained on a model CHI600A electrochemical analyzer. The HOMO levels of the Eu complexes were calculated based on their oxidation potentials, while LUMO was calculated based on the HOMO energy level and the lowest-energy absorption edge of the UV-vis absorption spectrum. Synthesis of 5-Diethylamino-1,10-phenanthroline (DEP) [11] The preparation of DEP required two steps. (1) 1,10-phenanthroline-5,6-epoxide (0.40 g, 2.04 mmol) was dissolved in a mixture of water (20 mL) and diethylamine (20 mL). The reaction mixture was stirred under nitrogen at ambient temperature overnight. Water (20 mL) was added and the mixture was extracted with dichloromethane. The combined organic phase was dried with Na2CO3 and then concentrated on a rotary evaporator. The crude product was further purified by chromatography to give of the desired pure product 5-diethylamino-5,6-dihydro-6-hydroxy-1,10-phenanthroline (0.48 g, 86%). (2) To a suspension of NaH (60%, 0.8 g) in dry THF (40 mL), 5-diethylamino-5,6-dihydro-6-hydroxy-1,10-phenanthroline (0.48 g) of was added. The mixture was refluxed under nitrogen for 4 h. Methanol (10 mL) was then added slowly to quench the excess NaH. The solvents were evaporated in vacuum and the product was purified by silica gel column using hexane/dichloromethane (1/3) as a eluent to give 5-diethylamino-1,10-phenanthroline as a brownish oil (90%, 0.38 g). 1H NMR (CDCl3, δ): 1.07 (t, J = 6.8 Hz, 6 H), 3.22 (q, J = 6.8 Hz, 4 H), 7.22 (s, 1 H), 7.48 (dd, J = 8.0, 8.0 Hz, 1 H), 7.58 (dd, J = 8.0, 8.0 Hz, 1 H), 8.05 (d, J = 8.0 Hz, 1 H), 8.61 (d, J = 8.0 Hz, 1 H), 9.00 (d, J = 8.0 Hz, 1 H), 9.11 (d, J = 8.0 Hz, 1 H). HRMS (m/z): calcd for C16H17N3 251.1423, found 251.1422. 5-Piperidine-1,10-phenanthroline (PiPhen) The procedure used for the synthesis of PiPhen is similar to that for DEP and piperidine (20 mL) instead of diethylamine was used. The yields for steps 1 and 2 were 82% and 85%, respectively. 1H NMR (CDCl3, δ): 1.60 - 1.82 (m, 6 H), 3.04 (t, J = 6.8Hz, 4 H), 7.18 (s, 1 H), 7.51 (dd, J = 8.0, 8.0 Hz, 1 H), 7.59 (dd, J = 8.0, 8.0 Hz, 1 H), 8.07 (d, J = 8.0 Hz, 1 H), 8.53 (d, J = 8.0 Hz, 1 H), 9.00 (d, J = 8.0 Hz, 1 H), 9.12 (d, J = 8.0 Hz, 1 H). HRMS (m/z): calcd for C17H17N3 263.1422, found 263.1422. General Procedure for the Synthesis of Europium Complexes [13] To a 25-mL side-arm flask under nitrogen were added EtOH (10 mL), β-diketone (3 mmol) and triethylamine (3 mmol). After 10 min, to the flask was added phenanthroline derivative (1 mmol), then EuCl3 (1 mmol) was injected to the flask dropwise. The reaction mixture was heated and stirred at 50 ~ 60 °C under nitrogen for an appropriate time (3 ~ 5 h). The reaction mixture was then cooled to room temperature. The solvent was removed under reduced pressure and the residue was washed with water. The crude product was then recrystallized by 80% ethanol to afford the desired product. The product was purified further by sublimation at 4 ~ 6 ×10-3 Pa. Product yield, melting point and elemental analysis data are listed below. Eu(TTA)3(DEP) (Eu-DEP). Yield: 83%. Mp. 177oC. Anal. calcd. C: 45.03, H: 2.74, N: 3.94, found C: 44.83, H: 2.72, N: 3.80. Eu(TTA)3(PiPhen) (Eu-PiPhen). Yield: 80%. Mp. 162oC. Anal. calcd. C: 45.65, H: 2.71, N: 3.89, found C: 45.78, H: 2.90, N: 3.88. Results and Discussion Synthesis of Europium Complexes Aminophenanthroline derivatives were successfully prepared via a three-step process as shown in Figure 1. 1, 10-Phenanthroline was first oxidized to a 1, 10-phenanthroline-5,6-epoxide which then reacted with an amino compound to give the corresponding 5-amino-6-hydroxy-phenanthroline. Dehydration of the hydroxy compound afforded the desired product in 70~77% yields (Scheme 1). Europium complexes were then prepared from EuCl3, 2-thenoyltrifluoroacetone (TTA), the corresponding phenanthroline derivative in the presence of triethylamine in ethanol at 50ºC in 80~83% yields (Scheme 2). These europium complexes were further purified by vacuum sublimation (220ºC, 5x10-5 Torr) before device fabrication. Physical Properties of Europium Complexes The UV absorption and PL data of the europium complexes (Eu-DEP and Eu-PiPhen) are summarized in Table 1. The UV and PL spectra of these complexes in dichloromethane solutions are revealed in Figure 2. In spite of the difference of the substituent on the phenanthroline ligands, these two complexes show the same peaks of absorption and emission maximums. These two europium compounds emit a strong sharp red band at ~612 nm in solution and in the solid state. This band is a characteristic Eu3+ emission due to the transition 5D0→7F2 of the central europium metal ion [9]. The highest occupied molecular orbital (HOMO) and lowest unoccupied molecular orbital (LUMO) of these europium complexes were determined and listed in Table 1. The HOMO energy of these products are at ca ~ 5.6 eV much higher than those reported for most europium complexes. The results indicate that the amino groups in these two complexes influence greatly the HOMO levels of the complexes as expected. 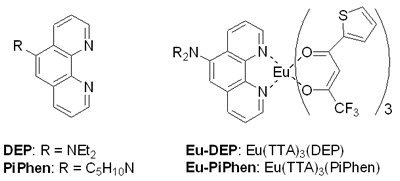 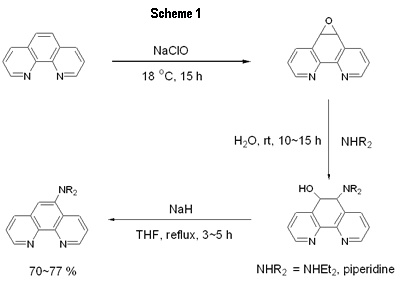 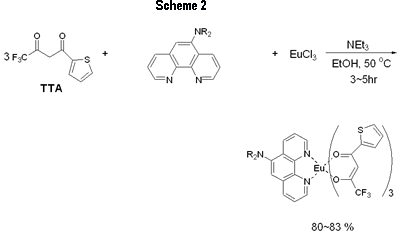 | Figure 1. Chemical structure of the phenanthroline ligand and europium complexes. | 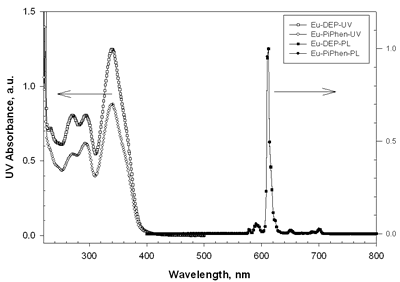 | Figure 2. The UV-vis absorption and PL spectra of Eu-DEP and Eu-PiPhen in CH2Cl2 solution. | Table 1. Physical properties of europium complexesa | Eu-DEP | 270 (80700), 294 (80600), 340 (125000) | 612 | 5.6 | 2.7 | Eu-PiPhen | 272 (54500), 294 (61510), 340 (87600) | 612 | 5.6 | 2.7 | aMeasured in a CH2Cl2 solution. bThe excitation wavelength is 380 nm. Electroluminescent Devices Based on Europium Complexes To understand the efficiency of the europium complexes as red emitters in electroluminescent devices, several devices were fabricated. Figure 3 shows the general configuration of devices with europium complex doped into CBP. TPD (4,4’-bis[N-(p-tolyl)-N-phenyl-amino]biphenyl) or NPB (4, 4 – bis [N - (1 - naphthyl)-N-phenylamino] biphenyl) is used as the hole transporting layer with thickness 50 nm. A europium complex doping in CBP or TPBI (1,3,5 - tris [ N – phenylbenzimidazol -2-yl]benzene) is the emitting layer, while BCP is served as a hole blocker. The electron transporter is Alq (tris[8-hydroxyquinoline]) or TPBI. The detailed device structures and key characteristics of these devices are shown in Table 2. 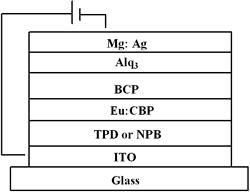 | Figure 3. The general device configuration with europium complex doped into CBP. | Table 2. Performance of Eu-Based OLEDsa | A | 4.7 | 0.52, 7.4 | 779, 12.4 | 0.96, 7.4 | 0.41, 7.4 | (0.59, 0.32) | B | 4.6 | 0.83, 6.6 | 555, 12.6 | 1.62, 6.6 | 0.77, 6.6 | (0.61, 0.32) | C | 6.3 | 1.74, 8.6 | 751, 16.8 | 3.45, 8.6 | 1.26, 8.6 | (0.65, 0.33) | D | 6.0 | 0.68, 10.2 | 767, 15.8 | 1.31, 10.2 | 0.41, 9.8 | (0.61, 0.33) | E | 7.3 | 0.16, 11.2 | 249, 16.0 | 0.30, 11.2 | 0.09, 10.8 | (0.64, 0.34), 10V | F | 8.1 | 0.14, 13.2 | 221, 18.6 | 0.28, 13.2 | 0.07, 13.2 | (0.64, 0.34), 10V | aThe data for external quantum efficiency (ηext), brightness (L), current efficiency (ηc) and power efficiency (ηp) are the maximum values of the device. bA: TPD (50 nm)/Eu-DEP:CBP(4.5%, 30 nm)/BCP (30 nm)/Alq (25 nm); B: TPD (50 nm)/Eu-DEP:CBP (7.2%, 30 nm)/BCP (30 nm)/Alq (25 nm); C: NPB (50 nm)/Eu-PiPhen:CBP (5.3%, 30 nm)/BCP (30 nm)/Alq (25 nm); D: NPB (50 nm)/Eu-PiPhen:CBP (7%, 30 nm)/BCP (30 nm)/Alq (25 nm). E: NPB (20 nm)/CBP (20 nm)/Eu-DEP:TPBI (9.6%, 40 nm)/TPBI (10 nm); F: NPB (20 nm)/CBP (20 nm)/Eu-PiPhen:TPBI (10%, 40 nm)/TPBI (10 nm). Device A and B using Eu-DEP having diethylaminophenanthroline as the dopant had the same device configuration of ITO/TPD/Eu-DEP:CBP/BCP/Alq but different dopant concentration 4.5 and 7.2%. The EL spectra of device A exists trace emission line at ~ 400 nm and strong red emission of Eu3+ at 612 nm (Figure 4(a)). Increasing the concentration of Eu-DEP to 7.2% efficiently quenches the 400-nm emission (Figure 4(b)). The CIE coordinates of device B calculated based on the EL data are (0.61, 0.32). The luminance and current density versus voltage characteristics of these devices is displayed in Figure 5. The detailed electroluminescent data are also shown in Table 2. The maximum brightness of device A and B are 779 and 555 cd/m2, respectively. An external quantum efficiency 0.83%, current efficiency 1.62 cd/A and power efficiency 0.77 lm/W were obtained from device B. ![AZoJoMo - AZoM Journal of Materials Online - The EL spectra of device A [TPD (50 nm)/Eu-DEP:CBP (4.5%, 30 nm)/BCP (30 nm)/Alq (25 nm)] at applied potential 5 V ~ 17 V.](/work/kWfhsWyNQW0iTbit6cl5_files/image006.gif) ![AZoJoMo - AZoM Journal of Materials Online - (b) The EL spectra of device B [TPD (50 nm)/Eu-DEP:CBP (7.2%, 30 nm)/BCP (30 nm)/Alq (25 nm)] at applied potential 5 V ~ 17 V](/work/kWfhsWyNQW0iTbit6cl5_files/image007.gif) | Figure 4. (a) The EL spectra of device A [TPD (50 nm)/Eu-DEP:CBP (4.5%, 30 nm)/BCP (30 nm)/Alq (25 nm)] at applied potential 5 V ~ 17 V. (b) The EL spectra of device B [TPD (50 nm)/Eu-DEP:CBP (7.2%, 30 nm)/BCP (30 nm)/Alq (25 nm)] at applied potential 5 V ~ 17 V. | 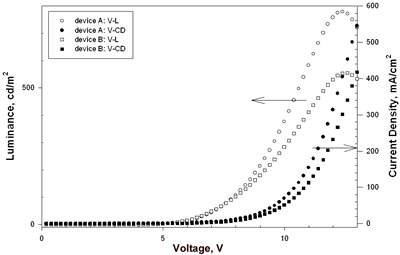 | Figure 5. The luminance and current density versus voltage characteristics of device A and device B. | Eu-PiPhen having the similar aminophenanthroline ligand as Eu-DEP was also used to fabricate devices. Device C and D had two dopant concentration 5.3 and 7% of Eu-PiPhen and used NPB as hole transporter, CBP as host, BCP as hole blocker and Alq as electron transporter. The device performance and configuration are shown in Table 2. The EL spectra of devices C and D show almost pure red light emission at applied potential of 6 to 16 V (Figure 6). The CIE coordinates calculated by the EL data at 8 V for these two devices are (0.65, 0.33) and (0.61, 0.33), respectively. The CIE coordinates of device C appeared at the position of the NTSC standard red (x = 0.65, y = 0.33). This CIE coordinates are among the best reported for devices incorporating a europium complex as a red emitter. The luminance and current density versus voltage characteristics of these devices is displayed in Figure 7. The electroluminescent data extracted from Figure 7 are listed in Table 2. Devices C and D exhibit similar maximum brightness, 751 and 767 cd/m2, respectively, but device C shows better efficiency compared to device D. The external quantum efficiency of 1.74%, current efficiency of 3.45 cd/A and power efficiency of 1.26 lm/W for device C are the highest among these devices. ![AZoJoMo - AZoM Journal of Materials Online - The EL spectra of device C [NPB (50 nm)/Eu-PiPhen:CBP (5.3%, 30 nm)/BCP (30 nm)/Alq (25 nm)] at applied potential 6 V ~ 16 V.](/work/kWfhsWyNQW0iTbit6cl5_files/image009.gif) ![AZoJoMo - AZoM Journal of Materials Online - The EL spectra of device D [NPB (50 nm)/Eu-PiPhen:CBP (7%, 30 nm)/BCP (30 nm)/Alq (25 nm)] at applied potential 6 V ~ 16 V.](/work/kWfhsWyNQW0iTbit6cl5_files/image010.gif) | Figure 6. (a) The EL spectra of device C [NPB (50 nm)/Eu-PiPhen:CBP (5.3%, 30 nm)/BCP (30 nm)/Alq (25 nm)] at applied potential 6 V ~ 16 V. (b) The EL spectra of device D [NPB (50 nm)/Eu-PiPhen:CBP (7%, 30 nm)/BCP (30 nm)/Alq (25 nm)] at applied potential 6 V ~ 16 V. | 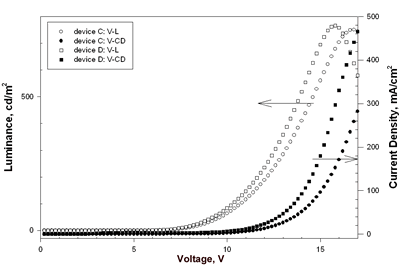 | Figure 7. The luminance and current density versus voltage characteristics of device C and device D. | TPBI was also chosen as a host for doping europium complexes in the EL devices. It is noteworthy that the emission spectrum of TPBI is very similar to that of CBP (Figure 8). In addition, the wide energy gap of TPBI covers the HOMO and LUMO levels of europium complexes. Devices E-F with the device structure [NPB (20 nm)/CBP (20 nm)/Eu:TPBI (x%, 40 nm)/TPBI (10 nm)], where the europium complexes = Eu-DEP and Eu-PiPhen, were made. 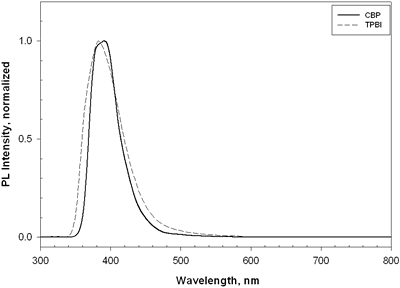 | Figure 8. The PL spectra of CBP and TPBI thin films. | Device E consisting of 9.6% Eu-DEP in TPBI shows only the characteristic red emission of Eu+3 at 612 nm. Device F using Eu-PiPhen as the dopant with the same device configuration as that of device E shows the same emission characters. For both devices, the EL spectra do not change at applied potentials of 10 ~ 14 V. The CIE coordinates calculated from the EL data are (0.64, 0.34) very close to that of the NTSC standard red (x = 0.65, y = 0.33), but the maximum brightness for these two devices are just more than 200 cd/m2. The detailed data for these devices are also listed in Table 2. An EL device with device structure similar to that of device C but using Eu(TTA)3(Phen) (Eu-Phen) as the dopant emitter was fabricated for comparison. This device emits, in addition to the red light at 612 nm from the Eu center, a small amount of light from CBP and Alq with maxima at ca 400 and 510 nm, respectively. The portion of light emitted form CBP and Alq increases with increasing operational voltage. The CIE coordinates at 8 V is (x = 0.59, y = 0.33) and external quantum efficiency and current efficiency are 1.50% and 2.91 cd/A, respectively, for this device. Thus, the devices based on Eu-DEP and Eu-PiPhen appear to emit more saturated red light than the device using Eu-Phen as the dopant emitter. The presence of an amino group on the phenanthroline ring of complexes Eu-DEP and Eu-PiPhen is likely responsible for this observation. Eu-DEP and Eu-PiPhen show HOMO levels at 5.6 eV ca. 0.4 eV higher than the values for other europium complexes reported that do not have an electron donating group on the phenanthroline ring. The HOMO and LUMO levels of these two europium complexes are in between the HOMO and LUMO levels (5.9 and 2.4 eV, respectively) of CBP molecules which serve as the host material for devices A-D. The holes and electrons in the CBP layer are readily trapped by the europium complexes that serve as dopant emitters leading to emission from the europium dopants. For europium complexes with their HOMO levels appearing at ~ 6 eV, the hole and electron trapping by these complexes are less favorable when these complexes are doped in the CBP layer due to the fact that the HOMO of CBP is higher than those of the europium complexes. The hole-electron recombination occurs not only on the Eu-Phen molecules, but also on the CBP molecules and on the Alq layer. The observation of light emission from the CBP and Alq layers in addition to the red emission from Eu-Phen for the Eu-Phen-based device can be attributed to the low HOMO level of complex Eu-Phen. Conclusion We synthesized two new europium complexes consisting of an electron-donating substituent on the phenanthroline ligand. These complexes emit characteristic red light strongly both in solution and in the solid state and were used as red dopant emitters in electroluminescent devices. Maximum brightness more than 700 cd/m2 and excellent CIE value were achieved for some europium-based devices. A Eu(TTA)3(PiPhen)-based device exhibits CIE coordinates of (0.65, 0.33) at the point of the NTSC standard red and shows external quantum efficiency of 1.74%, current efficiency of 3.45 cd/A and 1.26 lm/W. The present results indicate that the design of new ligands is crucial to enhance performance of electroluminescent devices based on rare-earth metal complexes. Acknowledgements We thank the Ministry of Education (grant no. 89-FA04-AA) for the support of this research. References 1. C. Adachi, M. A. Baldo, S. R. Forrest, S. Lamansky, M. E. Thompson and R. C. Kwong, “High-efficiency Red Electrophosphorescence Devices”, Appl. Phys. Lett., 78 (2001) 1622-1624. 2. H. Heil, J. Steiger, R. Schmechel and H. von Seggern, “Tris(dibenzoylmethane) (monophenanthroline) Europium (III) Based Red Emitting Organic Light Emitting Diodes”, J. Appl. Phys., 90 (2001) 5357-5362. 3. J. Kido, H. Hayase, K. Hongawa, K. Nagai and K. Okuyama, “Bright Red Light-Emitting Organic Electroluminescent Devices having a Europium Complex as an Emitter”, Appl. Phys. Lett., 65 (1994) 2124-2126. 4. C. J. Liang, D. Zhao, Z. R. Hong, D. X. Zhao, X. Y. Liu, W. L. Li, J. B. Peng, J. Q. Yu, C. S. Lee and S. T. Lee, “Improved Performance of Electroluminescent Devices Based on an Europium Complex”, Appl. Phys. Lett., 76 (2000) 67-69. 5. “Electroluminescence Mechanisms in Organic Light Emitting Devices Employing a Europium Chelate Doped in a Wide Energy Gap Bipolar Conducting Host”, C. Adachi, M. A. Baldo and S. R. Forrest, J. Appl. Phys., 87 (2000) 8049-8055. 6. M. Noto, K. Irie and M. Era, “Efficient Red Electroluminescence from New Europium Complex”, Chem. Lett., 4 (2001) 320-321. 7. Z. Hong, C. Liang, R. Li, W. Li, D. Zhao, D. Fan, D. Wang, B. Chu, F. Zang, L. S. Hong, S. T. Lee, “Rare Earth Complex as a High-Efficiency Emitter in an Electroluminescent Device”, Adv. Mater., 13 (2001) 1241-1245. 8. J. Kido and Y. Okamoto, “Organo Lanthanide Metal Complexes for Electroluminescent Materials”, Chem. Rev., 102 (2002) 2357-2368. 9. O. L. Malta, H. F. Brito, J. F. S. Menezes, F. R. Goncalves e Silva, C. de Mello Donega and S. Alves Jr., “Experimental and Theoretical Emission Quantum Yield in the Compound Eu(thenoyltrifluoroacetonate)3.2(dibenzyl sulfoxide)”, Chem. Phys. Lett., 282 (1998) 233-238. 10. P. P. Sun, J. P. Duan, H. T. Shih and C. H. Cheng, “Europium Complex as a Highly Efficient Red Emitter in Electroluminescent Devices”, Appl. Phys. Lett., 81 (2002) 792-794. 11. Y. B. Shen and B. P. Sullivan, “A Versatile Preparative Route to 5-Substituted-1,10-Phenanthroline Ligands Via 1,10-Phenanthroline 5,6-Epoxide”, Inorg. Chem., 34 (1995) 6235-6236. 12. D. Malacara, “Color Vision and Colorimetry: Theory and Applications”, SPIE-The International Society for Optical Engineering, Bellingham,Washington U.S.A. (2002). 13. L. R. Melby, N. J. Rose, E. Abramson and J. C. Laris, “Synthesis and Fluorescence of Some Trivalent Lanthanide Complexes”, J. Am. Chem. Soc., 86 (1964) 5117-5125 Contact Details |