Introduction Molecular imprinting is an effective method to create selective recognition sites in synthetic polymers [1]. As tailor-made polymer materials for recognition of a template molecule, molecularly imprinted polymers (MIPs) have been applied in chromatography [2], chiral compound separation [3], and solid phase extraction [4]. But little was known about the imprinted membrane with recognition functionality and binding ability to the target molecule. Kobayashi and colleagues have developed theophylline (THO) molecularly imprinted copolymer membranes using the phase inversion imprinting technique of poly (acrylonitrile-co-acrylic acid) [P (AN-co-AA)] (Scheme1) [5–8]. Thus far, phase inversion imprinting membranes have been made of various polymer materials for substrate permselective binding. In 2004, we successfully prepared molecularly imprinted poly (acrylonitrile-co-methylacrylic acid) [P(AN-co-MAA)] membranes for recognition and permselective binding of uracil (URA) (Scheme 2) [9]. URA selective binding is meaningful, since the subject is one application for bio-mimetic artificial components that compose RNA in biological organisms. Thus, it has great importance as a synthetic receptor to study permselective binding of URA by the imprinting membrane. Therefore, we have taken an interest in development of novel imprinting membranes that are targeted to URA, because their special properties are attractive in many fields especially in biology and medicine area [9, 10]. 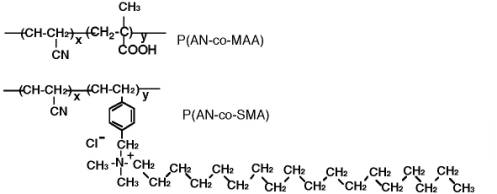 Scheme 1. Chemical structures of copolymers having MAA group (P(AN-co-MAA) and SMA group (P(AN-co-SMA). 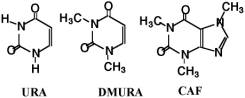 Scheme 2. Chemical structures of URA template and URA analogues of dimethylURA and Caffeine (CAF). So, in the present research, novel URA molecular imprinting membranes were studied by using ion complex method of copolymers, poly (acrylonitrile-co-methylacrylic acid) [P(AN-co-MAA)] and poly(acrylonitrile-co-vinylbenzyl-stearyldimethylamine chloride) [P(AN-co-SMA)] (Scheme 1). It is clarified that the ion complex technique provides a new approach in molecularly imprinted membranes. The clear difference in the present study and our previous studies is to apply two copolymers which can form ion complex membranes. Therefore, three dimensional molecule shapes for imprinting are encoded in the polymeric ion complex networks. It is our strategy for the URA imprinting that coagulated copolymers of methacrylic acid (MAA) and vinyl benzyl-stearyl dimethylamine (SMA) expect to form electrostatic networks to imprint the template molecule (Scheme 3). Besides, the quaternized ammonium group in the P(AN-co-SMA) also hydrophobically interacts with the template URA [10]. We would describe that URA imprinted membranes with different ionic components were prepared by phase inversion method. For their imprinted membranes, properties of resultant membranes were characterized in addition with both URA selectivity and binding nature. 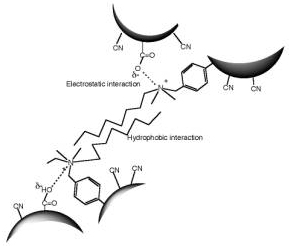 Scheme 3. Illustration of interactions between P(AN-co-MAA) having COO-group and P(AN-co-SMA) having positively charged quaternary ammonium group. Experimental Procedure Materials and Reagents Acrylonitrile (AN) was distilled twice in atmosphere and dimethyl sulfoxide (DMSO) and MAA were distilled twice under vacuum condition. We used URA (Beijing Fuxing Chemical Reagents Factory, Beijing, PRC), CAF (Farco Chemical Supplies, Hong Kong, PRC) and dimethyl uracil (DMURA) (Tokyo Kasei Co.) without purification for substrate binding experiments. All other reagents were used without further purification. Preparation of Membrane Materials As one of copolymer materials for the ion complexed membrane, P(AN-co-MAA) was synthesized with AN and MAA monomers (mole ratio AN:MAA=97.5:2.5) by radical polymerization [8]. For copolymerization of AN and MAA, DMSO and azobisisobutyronitrile were used as a polymerization solvent and initiator, respectively. The polymerization was carried out in the following manner under a nitrogen atmosphere. In a reaction vessel of 500 mL capacity, 28.8 g AN, 1.2 g MAA, 70 mL DMSO, and 0.153 g AIBN were mixed; then N2 gas was introduced. The copolymerization process was carried out at 60°C for 24 h. The resultant viscous mixture of P(AN-co-MAA) copolymer was precipitated in water and then washed with ethanol. The copolymer was obtained with 99% conversion after room temperature evacuation. The mole fraction of the MAA segments was determined as 10 mol% by IR method [7]. For another copolymer having SMA group, P(AN-co-SMA) was copolymerized with the same condition according to previous paper with 74.7% yield [11]. The mole fraction of the SMA segments in the copolymer was 10 mol% by analyzing with 400 MHz 1H-NMR (JNM GX 400 FT-NMR). Preparation of URA Imprinted Membranes by Phase Inversion Imprinting by Using P(AN-co-MAA) and P(AN-co-SMA) To imprint the URA molecule, copolymer membranes were obtained by ion complex of P(AN-co-MAA) and P(AN-co-SMA). Herein, different contents of their copolymers were used. To form the membranes, the cast solution containing both copolymers was prepared and phase inversion imprinting was applied according to previous reports [6, 8]. In DMSO-cast solvent, URA template and both P(AN-co-MAA) and P(AN-co-SMA) were dissolved with 10 wt% of total polymer concentration. The ratio of the copolymers in the solution was changed; P(AN-co-MAA):P(AN-co-SMA)= 0:10, 1:10, 1:5, 1:3, 1:2 and 1:1. Then, membrane was formed by using the DMSO solution in water for each mixed system. The resultant membrane was washed with 0.1 wt% acetic acid aqueous solution after the imprinting manner and then a large quantity of water was used to remove both URA and solvent. Membrane Properties and Binding Experiments Interaction between the copolymer membrane and URA template was studied by means of Fourier Transform Infra-Red Spectrometer (FT-IR, Prestige-21; Shimadzu Corp.). The morphological structure of URA imprinted membranes was observed using Scanning Electron Microscopy (SEM, JSM-5600; JEOL Co. Ltd.) and Atomic Force Microscopy (AFM, Nanopics 1000; Seiko Instruments, Inc.). AFM image analysis was performed with the Nanopics 1000 analysis program (Version 1.0); all images were analyzed with contacting mode using a silicon tip (DFM Cantilever-NPX1CTP004). Samples used in FT-IR spectrometry were prepared with a few micrometers thickness for transmittance mode (20 times integration). The sample membranes for SEM were prepared by fracturing membranes at temperature of liquid nitrogen and coating it with gold before measurement. Substrate binding experiments were carried out by batch experiment. For the batch experiment of URA solution or URA analog solution, the substrate concentration used was 32 μM. The concentration of the substrate in the solution was measured at different times by using UV-Vis (UV-2401; Shimadzu Corp.): The monitored wavelength was 259 nm for each molecule. Amounts of substrate binding were estimated before and after binding for the concentration and evaluated with mole binding per unit weight of imprinted polymer (μmol/g membrane). Results and Discussion Characterization of Copolymer Membranes Interaction between template molecule and membrane material plays an important role in molecular imprinting. In the present study, FT-IR was used to estimate the interaction between both copolymers. Figure 1 is FT-IR spectrum of P(AN-co-MAA), P(AN-co-SMA) and mixture of P(AN-co-MAA) and P(AN-co-SMA) with 1:1 ratio. From spectrum (a) of P(AN-co-MAA), characteristic peaks of C=O stretching at 1734 cm-1 and C-N stretching at 2243 cm-1 were found. It is known that the broad band near 3251-3547 cm-1 was caused by hydrogen bond interaction of COOH groups in the copolymer [7, 9]. In the spectrum (b) of P(AN-co-SMA), the quaternized ammonium group had characteristic peaks near 1639 and 3390 cm-1. Since the copolymer contained the phenylene group of the SMA segments, there were IR peaks at 750, 840, 870, and 910 cm-1. As shown in the spectrum (c) for the copolymers mixed with 1:1 ratio, the stretching bands of C=O at 1718 cm-1 and the quaternized ammonium at 1639 cm-1 were observed. Although the 1:1 mixture of both copolymers should contain the mixed spectrum of the corresponding (a) and (b), the observed peak of the C=O at 1734 cm-1 was shifted to 1718 cm-1 in the resultant (c) by formation of ion complex. Furthermore, it was noted in the spectrum that the new peak at 1028 cm-1 was observed in the mixed membrane. In addition, the spectral shape of the quaternary ammonium group near 3400 cm-1 region was different in both spectra of (b) and (c); The quaternized ammonium band was intense and shifted somewhat toward longer wavenumber side as the P(AN-co-SMA) was present with P(AN-co-MAA). This evidence suggested that their copolymers strongly interacted between the quaterinized ammonium group and the COOH group. That is, this indicated that both copolymers formed ion complex each other by electrostatic interactions between their functional groups (Scheme 3). Figure 1 has FT-IR spectrum (d) for URA imprinted membrane without extraction of the template, URA. The membrane was made of phase inversion process of both copolymers having URA; the mole ratio of [SMA] / [MAA] = 1/1. On the data the URA amide band overlapped with the 1629 cm-1 band, and slightly appeared at 1577 cm-1. It was noted that the ratio of the IR band intensity at 3394 cm-1 to that at 2931 cm-1, which was assigned by CH in the imprinted membranes, increased relative to that of unimprinted membrane. These evidences indicated that the URA template was present in the imprinted membrane. In the spectrum (a) of P(AN-co-MAA), the C=O band was observed at 1734 cm-1. We found that the IR peak appeared at 1721 cm-1 on the spectrum (d) was shifted to 1718 cm-1 in the (c) for the non-imprinted membrane. Here, the membrane was similarly prepared by using P(AN-co-MAA) and P(AN-co-SMA) without the template. Therefore, in the ion complexed membrane, the carbonyl groups of MAA interacted with the template. Also, the 1068 cm-1 band in the (b) for the quaternized ammonium group was shifted to 1028 cm-1 in the ion complex membrane (c). This was due to that ion complex was formed between the quaternized ammonium group and the COOH group in the non-imprinted membrane. But, as shown in the (d), the quaternized ammonium band was at 1078 cm-1, when the template was present. Similar tendency was observed in the IR band of 3427 cm-1 for the quarternized ammonium group. That is, the band was observed near 3394 cm-1 as the URA was added to the ion complex membrane. This is evidence that the URA molecule interacted with the SMA segments. 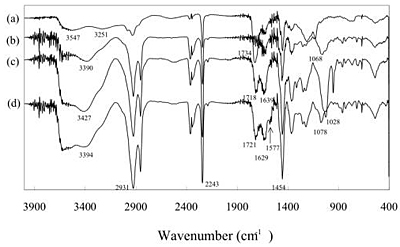 Figure 1. FT-IR spectra of P(AN-co-MAA) (a), P(AN-co-SMA) (b) and resultant on complex membranes prepared from the mixture solution containing both P(AN-co-MAA) and P(AN-co-SMA) without (c) and with (d) the URA template. The ratio of [COOH]/[SMA] was 1/1. Figure 2 presents SEM photographs of cross section of membranes made with different mixed ratio of P(AN-co-SMA):P(AN-co-MAA)=0:1 (a), 1:5 (b), 1:10 (c) for the imprinted membranes. For P(AN-co-SMA):P(AN-co-MAA)=0:1, it was shown that when there was no SMA segment with amphiphilic component in the membrane, the cross section of membrane presented a typical asymmetric porous structure. This indicated that quick solvent exchange between water and DMSO occurred and the coagulation of the copolymer was made [5, 12]. With increasing of the SMA component in the mixed membranes, the porous morphology in the resultant membrane turned to homogeneous one. It was observed that large pores in the cross section appeared when the ratio was changed from 1:5 to 1:10. However, the morphology at 1:10 showed the presence of both finger like macro-voids and sponge like layer in the same cross section of the resultant membrane. Note that the morphology of 1:10 was quite different to that of 0:1. Since the membrane morphology indicated different speed of the phase inversion of the copolymer from solution to solidify in water, the phase inversion speed became slow with increasing the amphiphilic SMA component in the ion complex membranes. In Figure 3, the observed SEM pictures were for unimprinted membarne (a) and imprinted membrane (b) made of P(AN-co-SMA):P(AN-co-MAA)=1:1. As presented, the unimprinted membrane showed somewhat porous structure with finger like layer, but the URA imprinted membrane showed dense and homogeneous structure in the cross section. The IR data of the 1:1 mixture of both copolymers suggested formation of ionically cross-linkable networks of both positively and negatively charged groups of the copolymers. It was considered from the dense morphology that the presence of URA template interfered the solvent exchange in the networks of the electrostatially charge interaction between the copolymers. This was also evidence that the URA template interacted with both copolymers through hydrogen bonding of MAA and hydrophobic interaction of SMA segments. 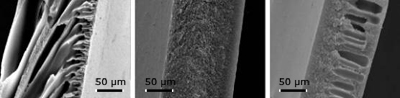 Figure 2. SEM photographs of cross section of membranes made with different mixed ratio of P(AN-co-SMA):P(AN-co-MAA)=0:1 (a), 1:5 (b), 1:10 (c) for the imprinted membranes. 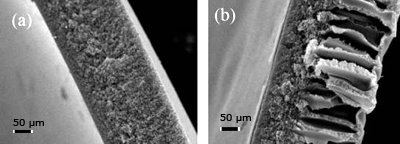 Figures 3. SEM pictures of imprinted membrane (a) and unimprinted (b) membrane made of P(AN-co-SMA):P(AN-co-MAA)=1:1. Figure 4 is AFM images of the resultant membranes made of P(AN-co-SMA):P(AN- co-MAA)=1:1 (a), 1:5 (b), 1:10 (c) with square size of 20x20 μm2. It could be observed that there was smooth surface of the whole membranes. But with increasing of MAA component in the ion complex membranes, the surface became somewhat rough. The values of average roughness of these surface were 4.8, 4.7 and 7.5 nm for the imprinted membranes prepared from 1:1, 1:3 and 1:5, respectively. This implied that the COOH groups in the MAA segments increased the phase inversion speed and made the resultant membrane surface rough. As presented in SEM, the dense morphology in the 1:1 membrane corresponded to the AFM data of (a). It was suggested in the data of AFM that the presence of the URA made the surface dense; The value of surface roughness was 120 nm for the surface of unimprinted membrane made of 1:1 ratio of the copolymers. 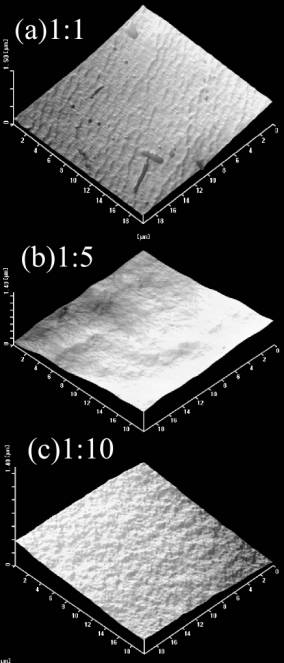 Figure 4. AFM images of the resultant membranes made of P(AN-co-SMA):P(AN-co-MAA)=1:1 (a), 1:5 (b), 1:10 (c) with square size of 20x20 µm2. URA Binding to the Imprinted Membranes Figure 5 is time course of URA binding amounts for each imprinted membrane made of P(AN-co-SMA):P(AN-co-MAA)= 0:10, 1:10, 1:2 and 1:1. It showed that all of the URA imprinted membranes had binding capacity to URA. The imprinted membrane prepared from P(AN-co-SMA):P(AN-co-MAA)=1:1 had the maximum URA binding capacity in this series. Figure 6 lists the saturate binding amount of URA in their imprinted membranes. Obviously, the tendency of high binding capacity to the URA was shown in P(AN-co-SMA):P(AN-co-MAA)= 1:1. 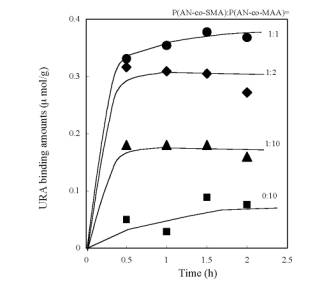 Figure 5. Time course of URA binding amounts for each imprinted membranes made of P(AN-co-SMA):P(AN-co-MAA)= 0:10, 1:10, 1:2 and 1:1. The URA concentration was 32 µM for the binding experiments. 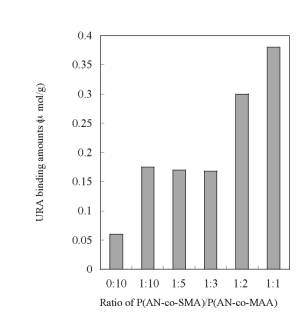 Figure 6. Values of saturate URA binding amount of URA imprinted membranes prepared from polymer cast solution containing P(AN-co-SMA):P(AN-co-MAA)= 0:10, 1:10, 1:5, 1:2 and 1:1. To check the selectivity of URA imprinted membrane prepared from the 1:1 mixture, batch experiment of DMURA and caffeine (CAF) binding were also examined. As shown in Scheme 2, DMURA and CAF contain similar chemical structure to URA template. But, the values of binding amounts of DMURA and CAF for the 1:1 membrane were about 0.06 μmol/g and were far less than that for template URA (Figure 7); The resultant value of [S]URA for the imprinted membrane was 0.38 μmol/g. Herein, we determined the values of the saturated binding amounts for URA ([S]URA), DMURA ([S]DMURA) and CAF ([S]CAF) at the equilibrium binding condition at 2 h. Also, the value of imprint factors αU/D was for the 1:1 imprinted membrane. Imprint factors of αU/D and αU/C were evaluated by the following formulas; for DMURA αU/D=[S]URA/[S]DMURA and for CAF αU/C=[S]URA/[S]CAF. So when the α>1, it means that resultant membrane has selective binding to URA. The resultant values of αU/D and αU/C were 6.3 for the imprinted membrane prepared from P(AN-co-SMA):P(AN-co-MAA)=1:1. The dense morphology of the imprinted membrane suggested that the dense structure of the ionically complexed membrane was useful for encoding the shape of the template molecule. 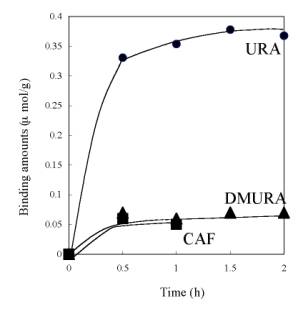 Figure 7. Comparison of time courses of banding amounts of DMURA and CAF with that of URA for the imprinted membrane prepared from P(AN-co-SMA):P(AN-co-MAA)= 1:1. Conclusions In summary, it was successfully demonstrated that polymer ion complex networks of P(AN-co-MAA) and P(AN-co-SMA) were used for URA imprinting. In phase inversion imprinting of the mixed copolymers, the polymeric networks were effective for formation of the imprinting membranes. The URA imprinted membranes were prepared by phase inversion imprinting of P(AN-co-MAA) and P(AN-co-SMA) with different components of both copolymers. FT-IR and SEM data showed that there was electrostatic interaction between both copolymers and gave the dense morphology of the imprinted membrane. It was concluded that the dense morphology of the imprinted membrane formed ion complex networks between the copolymers. This suggested an important role in the formation of the imprinted membrane. Acknowledgements We are grateful to Academic Frontiers Exchange Students Promotion Program in 2003 (No22) and The 21st century COE program, creation of hybridized materials with super functions and formation of an international research & education center of Nagaoka university of technology on Ministry of Education, Science, Sports, and Culture, Japan. This research was partially supported by the Grant-in-Aid for Scientific Research (B) (15310034) of the Japanese Ministry of Education, Science, Sports, and Culture and for Natural Science Fundation (004041200) and Henan Outstanding Youth Science Foundation. References 1. K. Mosbach, “Molecular imprinting”, Trends Biochem. Sci., Vol. 19, 9-14, 1994. 2. B. Sellergren, B. Ekberg and K. Mobach, “Molecular imprinting of amino acid derivatives in macroporous polymers: Demonstration of substrate- and enantio-selectivity by chromatographic resolution of racemic mixtures of amino acid derivatives”, J. Chromatogr., Vol. 347, 1-10, 1985. 3. L. Fischer, R. Muller, B. Ekberg and K. Mosbach, “Direct enantioseparation of .beta.-adrenergic blockers using a chiral stationary phase prepared by molecular imprinting”, J. Am. Chem. Soc., Vol. 113, 9358-9360, 1991. 4. A. Zander, P. Findlay, T. Renner and B. Sellergren, “Analysis of Nicotine and Its Oxidation Products in Nicotine Chewing Gum by a Molecularly Imprinted Solid-Phase Extraction”, Anal. Chem. Soc., Vol. 70, 3304-3314, 1998. 5. H. Y. Wang, T. Kobayashi and N. Fujii, “Molecular Imprint Membranes Prepared by the Phase Inversion Precipitation Technique”, Langmuir, Vol. 12, 4850-4856, 1996 6. H. Y. Wang, T. Kobayashi, T. Fukaya and N. Fujii, “Molecular Imprint Membranes Prepared by the Phase Inversion Precipitation Technique. 2. Influence of Coagulation Temperature in the Phase Inversion Process on the Encoding in Polymeric Membranes”, Langmuir, Vol. 13, 5396-5400, 1997. 7. T. Kobayashi, H.Y. Wang and N. Fujii, “Molecular imprint membranes of polyacrylonitrile copolymers with different acrylic acid segments”, Anal. Chem. Acta, Vol. 365, 81-88, 1998. 8. H. Y. Wang, T. Kobayashi and N. Fujii, “Surface molecular imprinting on photosensitive dithiocarbamoyl polyacrylonitrile membranes using photograft polymerization”,J. Chem. Tech. Biotechnol., Vol. 70, 355-362, 1997. 9. H. Y. Wang, S. L. Xia, H. Sun, Y. K. Liu, SH. K. Cao and T. Kobayashi, “Molecularly Imprinted Copolymer Membranes Functionalized by Phase InversionImprinting for Uracil Recognition and Permselective Binding”, J. Chromatogr., (B), Vol. 804, 127-134 , 2004. 10. S. L. Xia, H. Y. Wang and T. Kobayashi, “Phase Inversion Molecularly Imprinting of Uracil Targeted Membranes Made of Polyacrylonitrile Copolymers Having Methacrylic Acid and Acrylic Acid Segments for Recognition and Permselective Binding ”, Mater. Res. Soc. Proc. Boston, MA, 2003, Molecularly Imprinted Materials-2003, Vol. 787, 103-107, 2004. 11. T. Kobayashi, T. Nagai, H. Y. Wang and N. Fujii, “Charged ultrafiltration membranes of polyacrylonitrile covalently binding amphiphilic quaternary ammonium group: effect of aliphatic hydrophobic groups on the filtration properties” J. Membrane Sci., Vol. 112, 219-228, 1996. 12. R. M. Silverstein, C. G. Bassler and T. C. Morrill, “Spectrometric Identification of Organic Compounds [M]”, New York: John Wiley & Sons, 1991:118. 13. M. Mulder, “Basic principles of membrane technology”, Kluwer Academic Publishers, Netherlands, 1991. Contact Details |