Sponsored by XradiaApr 22 2013
Introduction - Magnesium Diboride
Magnesium diboride (MgB2) is recently recognized as a valuable superconducting material thanks to its high critical temperature. The potential applications of magnesium diboride include advanced MRI instruments, high-efficiency power transmitters, and next generation of electric motors.
Significance and Challenges of Characterization of Magnesium Diboride
In order to realize the aforementioned applications, it is essential to better understand the critical properties of magnesium boride. Intense research is being conducted into various processing techniques for optimization of the performance of magnesium diboride as a superconducting material by improving the in-field performance of its key current properties. Especially, malic acid doping is able to stabilize the critical current density of magnesium diboride as a function of applied field, although, the secret is yet to be fully revealed. Acquiring knowledge on this phenomenon holds potential for optimization of magnesium diboride and the performance properties of the final device by achieving more stable operating currents at higher applied fields.
However, the shortcomings of 2D microscopy techniques have limited the scope of research in this field as they able to show crack development in the structure but fail to provide adequate data to corroborate the degree of crack propagation. The data garnered through the use of transmission electron microscopy techniques is not sufficient to make definitive determinations of 3D morphology of superconducting materials.
Solution from Xradia
With high resolution and large field of view, the UltraXRM 3D X-ray microscope system from Xradia extends the scope of experimentation, such as microstructure response to stress. In a study conducted by Professor Satoshi Hata at Kyushu University, the objective was to analyze the impact of malic acid doping on the 3D microstructure of a magnesium boride superconducting tape so as to obtain 3D insight into change in superconductivity using the UltraXRM-L200 from Xradia.
Using 50 nm resolution, the UltraXRM-L200 was able to reveal several single- and sub-micron size scale cracks, as demonstrated in Figure 1. The penetration of the cracks into the sample material was appeared to be high, clearly demonstrating the reasons for nonlinearities in critical current density and variations in conductivity.
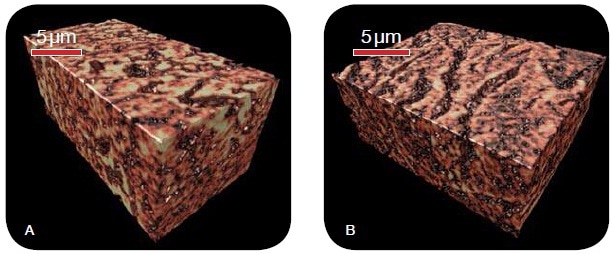
Figure 1. 3D microstructure of magnesium diboride, treated (A) with and (B) without malic acid doping, as imaged with the UltraXRM-L200 utilizing 50 nm resolution. The large cracks observed in the non-doped sample explain the observed differences in critical current density as a function of applied field between the two treatment schemes.
From the results, it was quantified that the pores in malic-acid doped samples were of larger average diameter and higher frequency when compared to the non-doped samples, thus leading to a reduction in superconductivity. The results of these measurements have been shown in Figure 2.
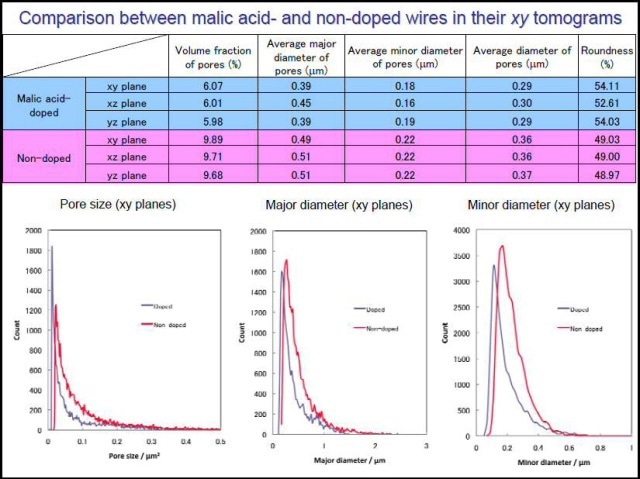
Figure 2. Quantitative microstructural analysis of the pore space shows the undoped sample has larger cracks, which leads to a reduction in superconductivity.
Conclusion
From the results, it is evident that the Xradia UltraXRM-L200 is a powerful tool for 3D characterization of magnesium diboride superconducting materials. Contrary to alternative techniques, the UltraXRM determines features and crack propagation using a resolution down to 50 nm and without affecting microstructure or compromising samples for further studies. Moreover, researchers can gain knowledge in 4D and beyond thanks to the non-destructive nature of this instrument, as it allows the variation of parameters such as heat and time in order to observe their effect on material microstructure.
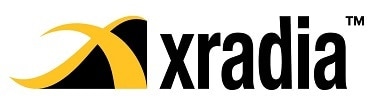
This information has been sourced, reviewed and adapted from materials provided by Xradia.
For more information on this source, please visit Xradia.