Aug 6 2019
Researchers from the Moscow Institute of Physics and Technology (MIPT) and elsewhere have modeled the behavior of a widely used lubricant under extreme conditions. Their calculations on Russian supercomputers spare the costly experiments and predict how the viscosity of 2,2,4-trimethylhexane changes between the standard conditions and a pressure as high as 10,000 times that in your room. The findings, reported in Fluid Phase Equilibria, are key for the industrial applications of similar fluids in aircraft engines, as fuel additives and electrical insulators.
Photo. Tenth Industrial Fluid Properties Challenge organizer Scott Bair (center), contest champion Marco A. Galvani Cunha (left) from Johns Hopkins University, and first runner-up Nikolay Kondratyuk from the Moscow Institute of Physics and Technology. (Image Credit: fluidproperties.org)
The study was recognized with a first-runner-up diploma at the 10th Industrial Fluid Properties Challenge held by the American Institute of Chemistry Engineers (AIChE), the American Chemical Society, and major companies.
Industry needs better fluid behavior models
Computer modeling of industrial fluids is an important alternative to real experiments, which are not always feasible. Not many laboratories can afford actual measurements at pressures as high as 10,000 atmospheres. That said, engineers working in the industry need to know how a lubricant behaves under such conditions, because they are a reality of modern aircraft engines and steam turbines (fig. 1).
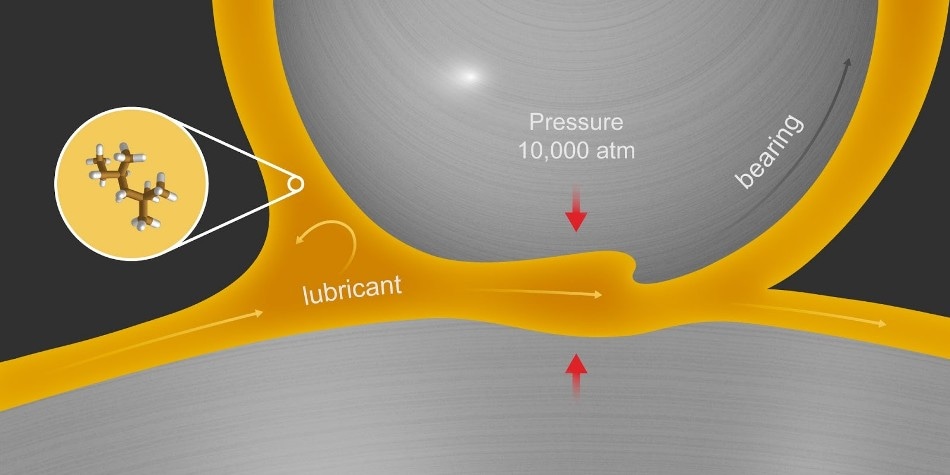
Figure 1. In a high-duty engine or turbine, lubrication reduces wearing by preventing a direct contact between the metal parts in a sliding contact bearing (depicted) or a gear train. Engineers need to know how the extreme pressures of up to 10,000 atmospheres affect a fluid’s viscosity, because once it reaches a certain critical value, it no longer provides adequate lubrication. Credit: @tsarcyanide/MIPT Press Office
“Computer modeling is appealing to companies, because it enables fast results by going over many possible options,” explained Nikolay Kondratyuk from the MIPT Laboratory of Supercomputing Methods in Condensed Matter Physics. “By rapidly testing hundreds of compound combinations in a simulation, one can design a lubricant. Rather than hire scores of researchers, the businesses find it financially more sound to fund contests where they can collect useful data on various models’ performance.”
Contests help select and perfect models
The Industrial Fluid Properties Challenge has researchers theoretically predict a certain property of some fluid important to the industry. This time it was about the shear viscosity of 2,2,4-trimethylhexane — a hydrocarbon used in motor oils — at pressures of up to 10,000 atmospheres. To determine the winners, the organizers ran an experiment and chose those simulations that mirrored reality the most.
The latest challenge, which ended in November, featured seven teams from the U.S. National Institute of Standards and Technology, Shanghai Jiao Tong University, Imperial College London, and elsewhere. The Russian team comprised Kondratyuk, the lead author of the competing paper, and co-author Vasily Pisarev, both affiliated with MIPT, the Joint Institute for High Temperatures of the Russian Academy of Sciences, and the Higher School of Economics.
“Every team had to submit a blind theoretical prediction, not knowing how the experiment turned out,” Kondratyuk commented. “Then, at the annual AIChE meeting in Pittsburg, Scott Bair revealed his experimental results and said we were only second to the Johns Hopkins team in terms of prediction accuracy.”
The viscosity values predicted by the Russian contestants for pressures between 1 and 5,000 atmospheres coincided with the experimental measurements within the error of the latter, or 3%. Beyond 5,000 atmospheres, the deviation of the model predictions gradually increases as the pressure goes up.
Computing power constrains simulations
Even a supercomputer is unable to model the behavior of lubricant molecules on timescales larger than a microsecond. This means that to obtain simulation results that are comparable to those measured in an experiment, the modeled data need to be extrapolated, or generalized beyond its original scope. There are two main options for doing this, associated with two distinct methods.
“We began by doing what every other team ended up doing. That is, we extrapolated the results of the nonequilibrium method,” Kondratyuk said. “But then we tested the equilibrium method, and it turned out viable across the entire pressure range. We ultimately submitted this second prediction, and it landed us in the second place.”
In addition to the benefits for the industry, testing the models of molecular interactions is of interest to fundamental science, since this research offers insights into the makeup of matter and how its particles interact.
The Russian team ran its calculations using the COMPASS model model of 1,000 interacting molecules and the supercomputers Desmos and Fisher at the Joint Institute for High Temperatures, RAS. The study was funded by the Russian Science Foundation.