A group of researchers from Italy developed an advanced smartphone-integrated magnifying lens with an adjustable plasmonic light-rejection filter.
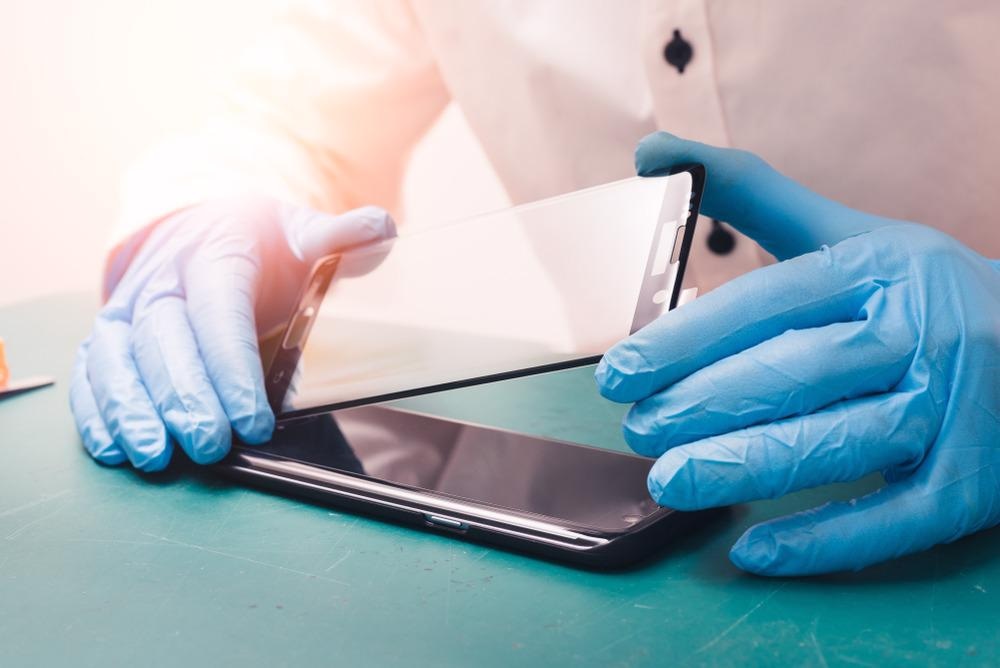
Study: 4D Printing of Plasmon-Encoded Tunable Polydimethylsiloxane Lenses for On-Field Microscopy of Microbes. Image Credit: Preechar Bowonkitwanchai/Shutterstock.com
The lens is fabricated by moldless 4D printing of polydimethylsiloxane (PDMS) polymer on nanosized-porous silicon (PSi) framework followed by in situ syntheses of nano-thick plasmonic filter on the surface of the lens using silver and gold nanoparticles (NPs). The smartphone integrated device showed promising real-world light filtering applications. This study is available in the journal Advanced Optical Materials.
What is a Plasmonic Rejection Filter?
Plasmonic nanoparticles are highly conductive metal or metal-like nanoparticles with a large number of free surface electrons/surface plasmons and negative permittivity in the optical frequency range showing a plasmonic effect.
In the plasmonic effect, electromagnetic waves such as visible light in the optical frequency range (from ultraviolet (UV) to near-infrared (NIR)) excite the surface plasmons and make them oscillate depending upon their wavelengths.
These inducible and tunable oscillations of surface plasmons in nanoparticles can be used to selectively absorb or scatter different wavelengths of light, which in turn depends upon the size, shape, composition, and density of nanoparticles.
Metals like silver (Ag), gold (Au), platinum (Pt), copper (Cu), and aluminum (Al), and a few natural ferroelectric materials have reportedly shown a plasmonic effect. A transparent film with well-dispersed plasmonic nanoparticles on its surface is called a plasmonic rejection filter.
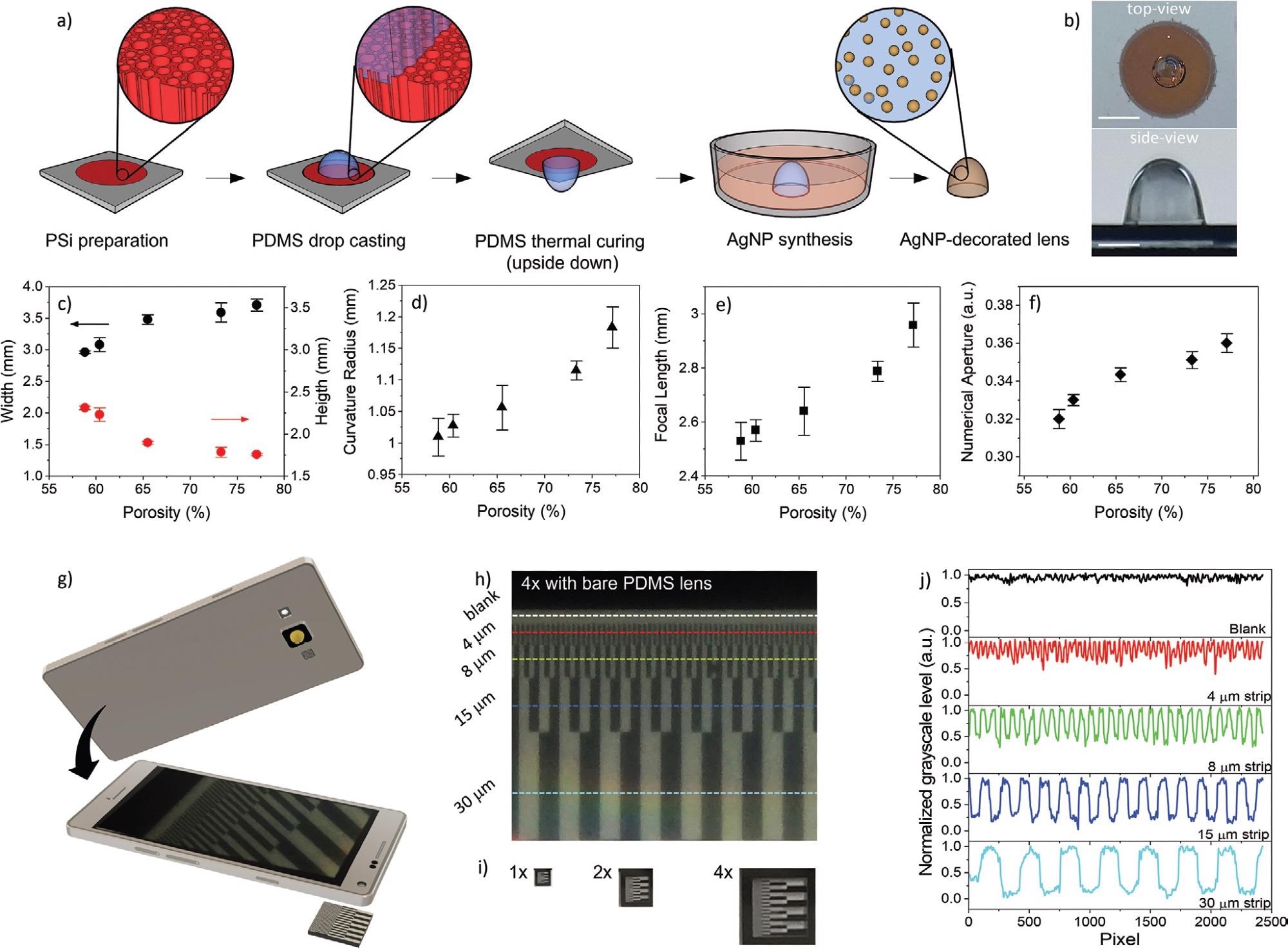
Preparation and characterization of PDMS lenses moldless-printed on a nanostructured porous silicon templating layer. a) Sketch of the moldless printing fabrication of a magnifying PDMS lens with different focal lengths from 2.5 to 2.8 mm, embedding an AgNP and/or AuNP plasmonic filter. b) Top-view and side-view of a PDMS lens standing on a PSi templating layer after upside-down curing. Markers are 5 mm (top) and 1 mm (bottom). c–f) Geometrical and optical properties of PDMS lenses (10 mg) prepared onto PSi templating layers with porosity of 58.8 ± 0.4%, 60.4 ± 0.1%, 65.5 ± 0.1%, 73.3 ± 0.5%, and 77.1 ± 0.2%, respectively. The error bars represent one standard deviation with respect to average values achieved over a number of 3 samples per porosity. g) Sketch of a smartphone coupled with a PDMS lens for imaging applications. h) Pictures of a chromium-on-quartz test pattern containing lines of width ranging from 250 to 4 µm with a constant duty cycle of 0.5, acquired with a PDMS lens with a focal length of 2.8 mm adhered to the camera of a commercial smartphone, at 4× digital zoom. i) Pictures of the test pattern in (h), captured with the as-received commercial smartphone using 1×, 2×, and 4× digital zoom. j) Grayscale intensity profiles of the lines in (h), from which it is apparent that lines with width down to 4 µm can be resolved (S/N ratio ≈10). Image Credit: Mariani, S et al., Advanced Optical Materials
About the Study
In this study, the researchers first fabricated a magnifying lens based on moldless 4D printing of PDMS on a PSi framework. PDMS is transparent, inert, nontoxic, and nonflammable, thus suitable as a lens material.
Additionally, PSi was a suitable material to accommodate a large volumetric change of PDMS during interaction with organic solvents. The upside-down thermal curing of the PDMS pre-polymer droplet on the PSi layer resulted in a PDMS lens with a prolate spheroid shape.
The swelling of 3D printed PDMS on PSi was reversible, which facilitated reversing of properties and functionalities of the prepared lens during the fabrication process, hence this process was termed as 4D printing. Then PDMS lenses were stripped from the PSi base layer to obtain the specimens.
Subsequently, a nano-thick plasmonic filter was synthesized on the surface of the PDMS lens by in situ fluoride-assisted depositions of Ag and Au NPs from AgF or HAuCl4:NH4F, respectively. The swelling of PDMS in ether and hexane solvent regulated the NP density per unit area on the surface of the lens, which in turn modulated the transmission of different wavelengths of light through it.
The prepared plasmon-encoded lens was integrated into a smartphone study of its real-world applications such as color tuning of a white light-emitting diode (LED), and bright-field and fluorescence microscopy to detect microbes in water.
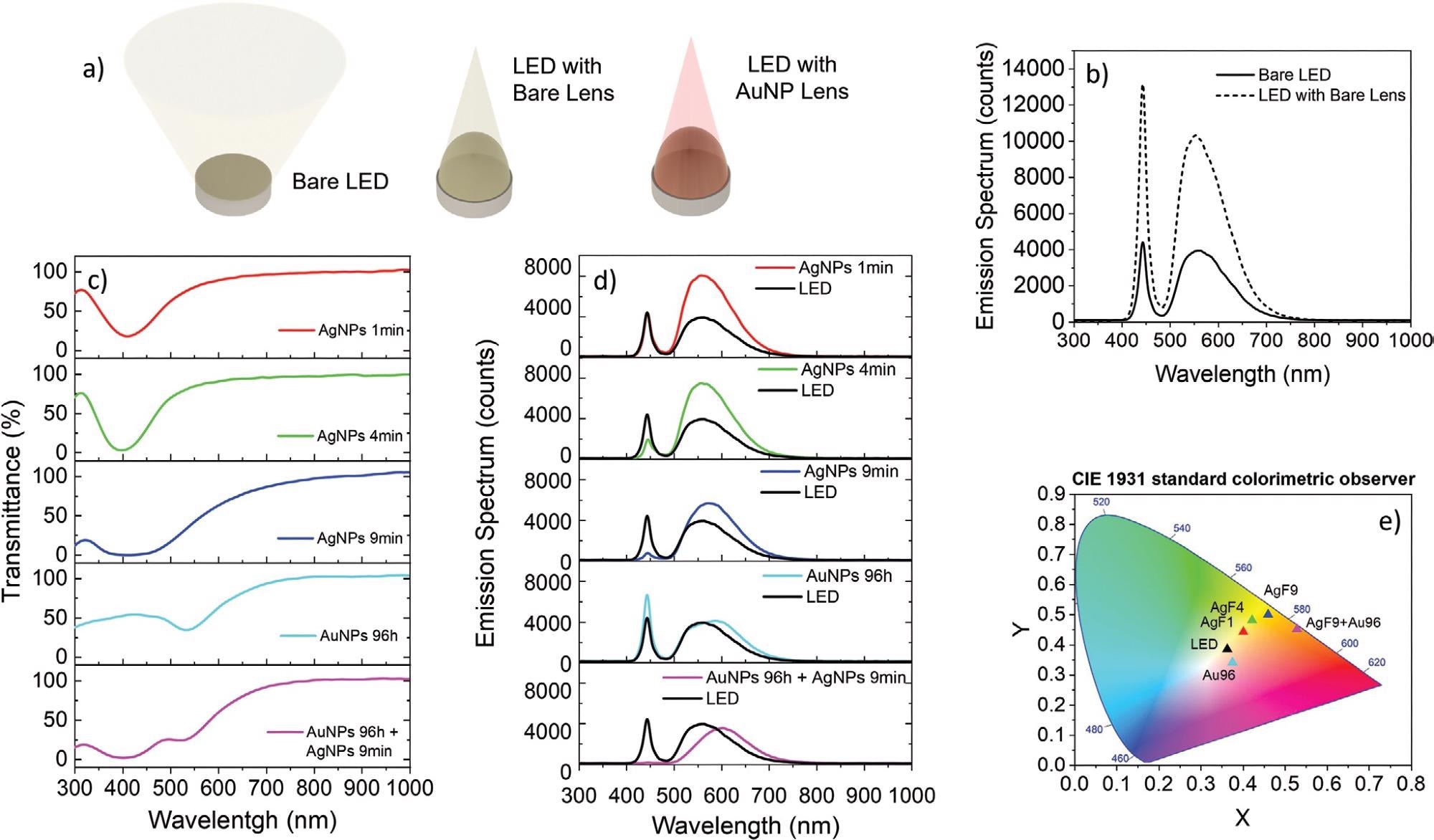
Lighting application of the PDMS lens decorated with AgNPs and/or AuNPs coupled to a white commercial LED. a) Sketch of the experimental configuration for the lighting application test. b) Emission spectra of a commercial white LED with/without a bare lens. c) Transmittance spectra of the PDMS lenses decorated with: AgNPs for 1, 4, and 9 min; AuNPs for 96 h; AgNPs for 9 min + AuNPs for 96 h. d) Emission spectrum of the bare white LED (black trace) superimposed to spectra of the LED coupled with PDMS lenses decorated with AgNPs and AuNPs as in (c). e) Spectral data from (d) represented in a CIE 1931 diagram of the color perceived by the human eye. Image Credit: Mariani, S et al., Advanced Optical Materials
Observations
Scanning electron microscopy (SEM) showed PSi had randomly arranged cylindrical pores with size and length of about 50 nm and 10 μm, respectively, and PDMS tuned the porosity from 59% to 77%. Also, uniform swelling ratios of about 8% and 10% were observed in the air after the treatment of the lens in diethyl ether and hexane, respectively
The PDMS lenses with and without NPs were placed on top of the LED of a smartphone and the light spectrums transmitted through the lenses were examined by a spectrometer.
The emission spectra indicated that the plasmonic filter encoded in the lens attenuated the intensity of the LED emission at specific wavelength intervals depending upon the localized surface plasmon resonance (LSPR), thus providing a simple and effective approach to tune the color of light transmitted through the lens.
Furthermore, an AgNP-decorated lens with a focal length of 2.8 mm was tested in fluorescence imaging applications by attaching it to the camera of a commercial smartphone, then the lens-smartphone system was used to image fluorescent polyethylene microbeads.
Moreover, the AgNP-decorated lens fully removed the excitation light thanks to the high optical density (OD) of 3 times at 403 nm wavelength, thus effectively retrieving the red auto-fluorescence of about 700 nm of the green chlorogonium sp. algal cells owing to the high extinction ratio (ER) of 60 dB.
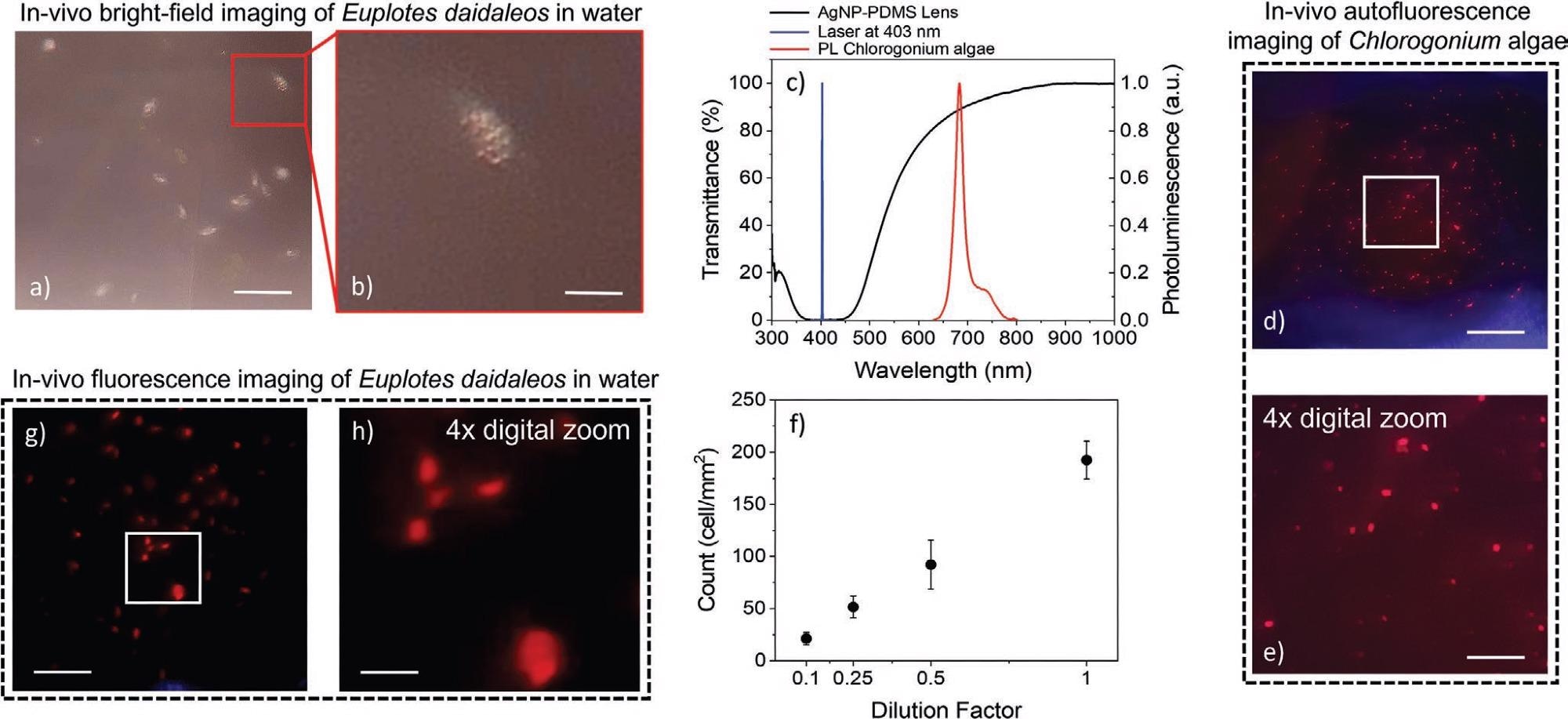
In vivo bright-field and fluorescence smartphone microscopy of auto-fluorescent unicellular microorganisms in a drop of water. a,b) Brightfield pictures at different magnifications of living Euplotes daidaleos in water acquired with a commercial smartphone equipped with a PDMS lens decorated with AgNPs for 9 min in an ethanolic solution of 15 × 10−3 m AgF. Scale bars in (a) and (b) are 200 and 50 μm, respectively. c) Transmission spectrum (black trace) of the AgNP-decorated PDMS lens (OD = 3, ER = 60 dB) and photoluminescence spectrum (red trace) of Chlorogonium algae excited with a blue laser at 403 nm (blue trace). d,e) Fluorescence pictures of Chlorogonium algae in water acquired with the lens-smartphone system, upon excitation with a blue laser at 403 nm. Scale bars in (d) and (e) are 200 and 50 μm, respectively. f) Cell density (cells mm−2) versus dilution factor of Chlorogonium algae as resulting from fluorescence images acquired using the lens-smartphone system. g,h) Fluorescence pictures of living Euplotes daidaleos after phagocytosis of Chlorogonium algae, acquired with the lens-smartphone system upon excitation with a blue laser at 403 nm. Scale bars in (g) and (h) are 200 and 50 μm, respectively. Image Credit: Mariani, S et al., Advanced Optical Materials
Conclusions
The researchers fabricated a PDMS-based lens by moldless 4D printing of PDMS pre-polymer on nanosized-porous silicon (PSi) framework followed by the synthesis of nano-thick plasmonic rejection filter on the surface of the lens using silver and gold nanoparticles (NPs).
The transmittance value of the plasmonic filter at the LSPR wavelength was pre-decided in the fabrication phase through controlled swelling and reverse swelling of the PDMS lens in hexane and ether solvents. Moreover, the integration of this lens with a smartphone camera provided a cost-effective, handheld, and field-portable solution for the spatiotemporal mapping of several microbes.
Reference
Mariani, S., Corsi, M., Paghi, A., La, A., Strambini, L., Frontini, F., Giuseppe, G., Barillaro, G., 4D Printing of Plasmon-Encoded Tunable Polydimethylsiloxane Lenses for On-Field Microscopy of Microbes. Adv. Optical Mater. 2021, 2101610. https://onlinelibrary.wiley.com/doi/epdf/10.1002/adom.202101610
Disclaimer: The views expressed here are those of the author expressed in their private capacity and do not necessarily represent the views of AZoM.com Limited T/A AZoNetwork the owner and operator of this website. This disclaimer forms part of the Terms and conditions of use of this website.