As electrical current flows through a battery, the materials within it gradually deteriorate. This degradation is influenced by various mechanical factors like stress and strain, although their exact effects on battery performance and lifespan remain only partially understood.
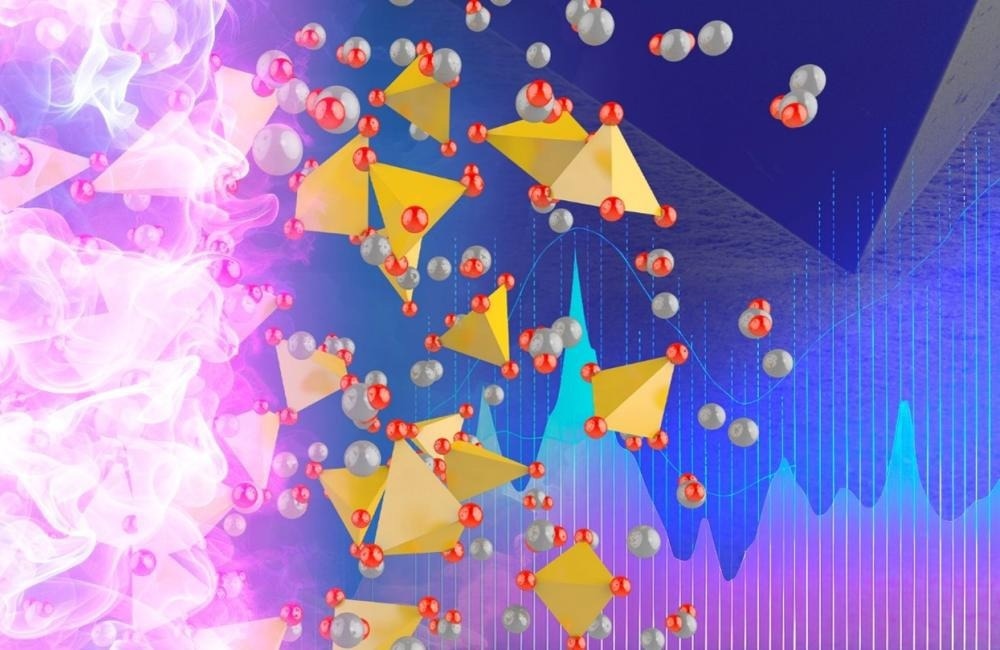
The image conceptualizes the processing, structure, and mechanical behavior of glassy ion conductors for solid state lithium batteries. Image Credit: Adam Malin/ORNL, US Department of Energy
A team of researchers, led by experts from the Department of Energy's Oak Ridge National Laboratory, has pioneered a framework for the design of solid-state batteries (SSBs) while taking these mechanical aspects into consideration. Their study, published in the journal Science, thoroughly examined the impact of these factors on SSBs as they undergo cycling.
Our goal is to highlight the importance of mechanics in battery performance. A lot of studies have focused on chemical or electric properties but have neglected to show the underlying mechanics.
Sergiy Kalnaus, Scientist, Multiphysics Modeling and Flows Group, Oak Ridge National Laboratory
The team, composed of experts from various ORNL research domains such as computation, chemistry, and materials science, collectively presented a more comprehensive understanding of the factors influencing SSBs by integrating insights from a diverse range of scientific perspectives. “We’re trying to bridge the divide between disciplines,” adds Kalnaus.
Batteries rely on charged particles moving through substances called electrolytes. While many electrolytes are in liquid form, as seen in lithium-ion batteries used in electric vehicles, there is ongoing development of solid electrolytes. These conductive materials are commonly constructed from glass or ceramics and have the potential to bring benefits such as improved safety and durability.
“True solid-state batteries don’t have flammable liquids inside,” notes Kalnaus. “This means that they would be less hazardous than the batteries commonly used today.”
Nonetheless, solid electrolytes are currently in their nascent stages of development due to the unique challenges associated with these materials. In solid-state batteries, the components experience expansion and contraction as they charge and transport mass, leading to changes in the overall system.
Electrodes constantly deform during the battery operation, creating delamination and voids at the interfaces with the solid electrolyte. In today’s systems, the best solution is applying a large amount of pressure to keep everything together.
Sergiy Kalnaus, Scientist, Multiphysics Modeling and Flows Group, Oak Ridge National Laboratory
The dimensional changes that occur can harm solid electrolytes, which are composed of brittle materials and are prone to breaking under strain and pressure. Enhancing the ductility of these materials would enable them to endure stress by flexing rather than fracturing. This characteristic can be achieved through certain methods that introduce minor crystal defects into ceramic electrolytes.
Electrons exit a system via anodes. In solid-state batteries, these anodes can be constructed from pure lithium, known as the most energy-dense metal. While this choice provides benefits in terms of battery power, it also generates pressure that has the potential to harm the electrolytes.
During charging, nonuniform plating and an absence of stress-relief mechanisms can create stress concentrations. These can support large amounts of pressure, enabling the flow of lithium metal. In order to optimize the performance and longevity of SSBs, we need to engineer the next generation of anodes and solid electrolytes that can maintain mechanically stable interfaces without fracturing the solid electrolyte separator.
Erik Herbert, Leader, Mechanical Properties and Mechanics Group, Oak Ridge National Laboratory
The team's efforts are a continuation of ORNL's extensive research into materials for SSBs. In the early 1990s, the lab pioneered the development of a glassy electrolyte called lithium phosphorous oxynitride, or LiPON. LiPON has gained widespread use as an electrolyte in thin-film batteries featuring a metallic lithium anode.
This component is capable of enduring numerous charge-discharge cycles without malfunction, primarily attributed to the ductility of LiPON. When subjected to mechanical stress, it exhibits a flowing response rather than developing cracks.
“In recent years we have learned that LiPON has robust mechanical properties to complement its chemical and electrochemical durability,” adds Nancy Dudney, an ORNL scientist who headed the group that developed the material.
The team's endeavor underscores an often-overlooked facet of SSBs, which is comprehending the elements influencing their longevity and performance.
Kalnaus concludes, “The research community needed a road map. In our paper, we outlined the mechanics of materials for solid-state electrolytes, encouraging scientists to consider these when designing new batteries.”