Larger K+ (ionic radius: 1.3 Å) can selectively permeate larger Na+ (ionic radius: 1.0 Å) through biological potassium ion channels, with selectivity ratios greater than 1000-fold. Scientists have been trying to replicate similar wonders in artificial systems for decades, but they have not been able to match the fine ion discrimination found in natural protein channels. Current artificial potassium channels typically have a K+/Na+ selectivity ratio of less than 40-fold.
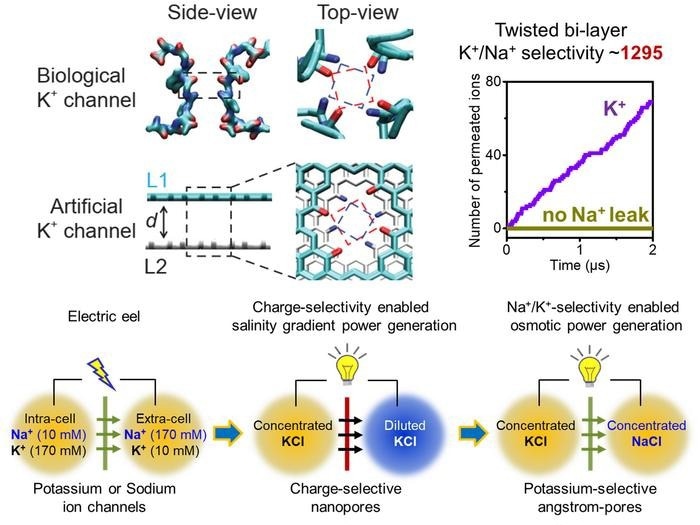
(Up) Molecular structure of the selectivity filter of a KcsA potassium channel. Adjacent carbonyl rings exhibit a 27-degree rotation. Inspired by this structural feature, bi-layer graphene angstrom-pores with twisted carbonyl rings are designed as artificial potassium channel, and strict Na+/K+ selectivity is found therein. (Down) the evolution of electric-eel-mimetic ionic power generation. Image Credit: Wei Guo
They discovered that the remarkable K+/Na+ selectivity derives from four periodic layers of carbonyl ring acting as the potassium ion binding sites by reexamining the atomic structure of the 12-Å-long four-fold symmetric tubular selectivity filter of biological KcsA potassium channels.
The two neighboring carbonyl rings do not exactly overlap, which is noteworthy. Instead, the dihedral angles of amino acids cause a rotation of over 27 degrees. The extremely strong K+/Na+ selectivity is supported by a structural property of the rotating carbonyl rings that has long been disregarded, but it has never been used to create artificial potassium channels.
In accordance with this design approach, the scientists grow angstrom-scale pores in bi-layer graphene sheets and embellish the two-pore margins with carbonyl group twisted rings. An artificial ion channel with a dynamic K+/Na+ selectivity ratio of up to 1295-fold can effectively block the transport of undesired Na+. The rate of K+ conduction is close to 3.5×107 ions/s or about 40% of the potassium ion channels found in biological systems.
The atomic trajectories of K+ permeation events reveal a dual-ion transport mechanism, which means that each successful K+ permeation must involve at least two potassium ions.
The release of a K+ from the bilayer nanopore exit is accomplished through a soft knock-on process from another K+ at the entrance, which has previously only been seen in biological ion channels. The formation of K-nH2O-K triplets by one or two hydrate water molecules between the graphene layers facilitates the synergetic action.
Besides providing new insights into the principles of biological systems, engineered ion channels with high selectivity for potassium allow for novel applications not possible in nature.
The researchers suggest a novel method of harvesting ionic power as a proof-of-concept demonstration: mixing electrolyte solutions of equal concentration through the bi-layer angstrom-pores. In theory, the biomimetic gadget may produce graphene sheets with less than 1% porosity and still attain a very high power density of around 1200 W/m2.
Notably, the osmotic power generation facilitated by potassium-permselectivity (PoPee-OPG) more closely resembles the manner in which the electrocyte cell of electric eels converts energy (see the IMAGE). First, solutions with the same total ionic strength could be used to run PoPee-OPG. In contrast, the salinity gradient power generation (SGPG) now in use is limited in its performance by the necessary diluted solutions.
It is important to note that because of the equilibrium of osmotic pressure across the cell membrane, there is not actually a low-concentration area in the body fluid of electric eels. The notable enhancement of PoPee-OPG is comparable to a big reduction in an ionic power source's internal resistance, hence permitting high output power. From this perspective, the electric-eel-mimetic energy conversion is improved by PoPee-OPG.
The article’s unique pore structure and operating principles, though still theoretical at this point, could guide the creation of highly selective membranes using materials such as stacked graphene or bi-layer covalent-organic framework components.
Overall, biomimetic design offers a blueprint for a variety of applications such as chemical separation, ionic batteries, water purification, and more by incorporating the remarkable properties of natural proteins into human-engineered systems.
Journal Reference
Li, J., et al. (2023) Designing artificial ion channels with strict K+/Na+ selectivity toward next-generation electric-eel-mimetic ionic power generation. National Science Review. doi:10.1093/nsr/nwad260