By Nidhi DhullReviewed by Lexie CornerUpdated on Aug 12 2024
Nanomaterials have become a significant force in modern science and industry, with applications in electronics, medicine, energy, food technology, telecommunications, and space exploration.1 Their tunable chemical, physical, and mechanical properties, along with superior performance compared to their bulk counterparts, enhance their importance in technological advancements.2
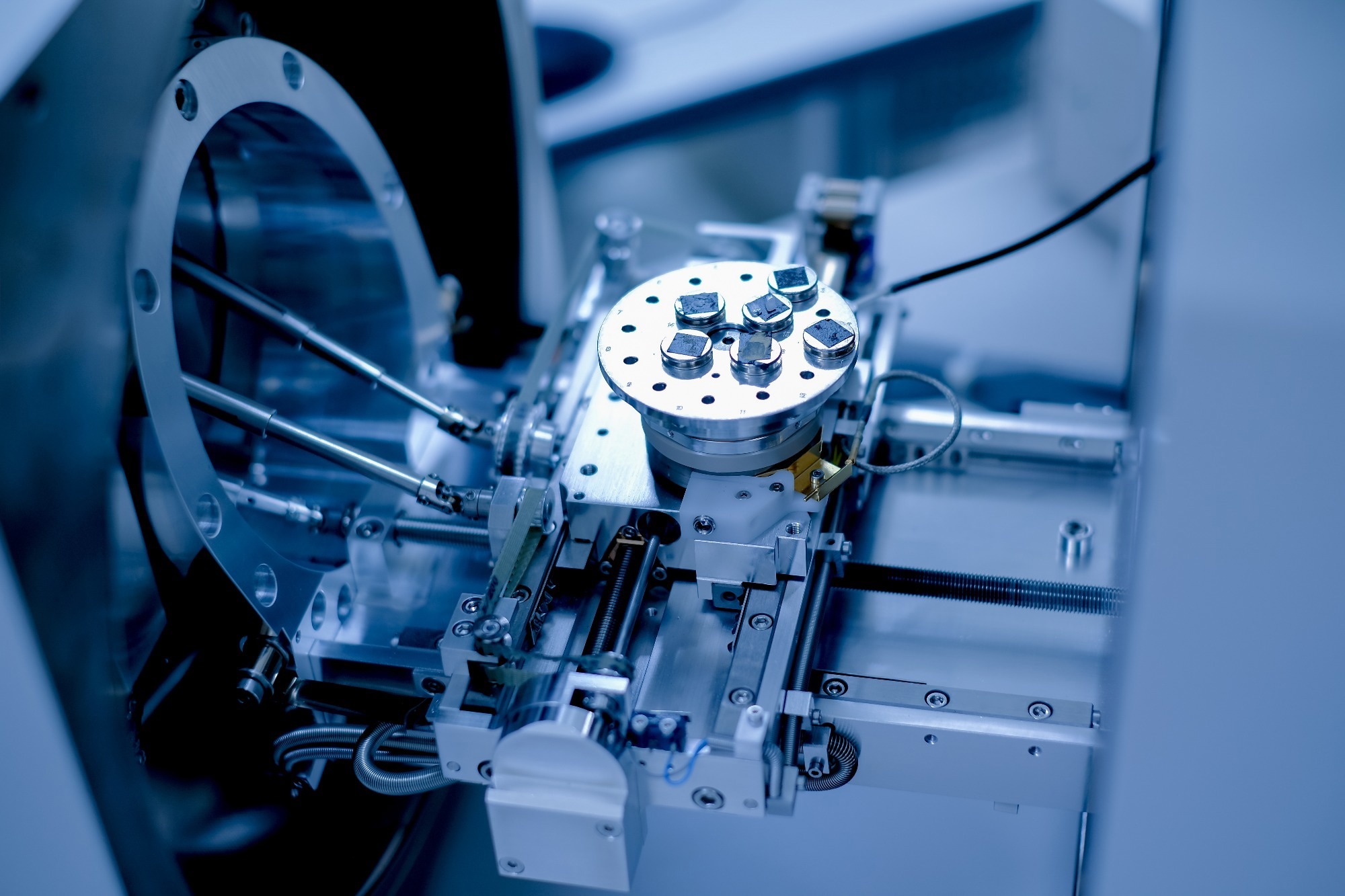
Image Credit: Anucha Cheechang/Shutterstock.com
What Are Nanomaterials?
Nanomaterials are characterized by having at least one dimension in the 1-100 nm range and a surface-to-volume ratio exceeding 60 m2/cm3. Unlike their bulk counterparts, the physicochemical properties of nanomaterials depend on their dimensions. Consequently, they exhibit unique thermal, chemical, magnetic, electric, optical, and physical properties influenced by their chemical composition.1
Mechanically, nanomaterials can enhance alloy hardness and ceramic super-plasticity.1 Their large surface area allows for modifications that improve mechanical properties such as hardness, adhesion, stress, strain, and elastic modulus.2
This also facilitates direct heat transfer on the surface, with surface atoms exhibiting high energy and a tendency to agglomerate.1,2 As a result, the melting temperatures of nanomaterials decrease with decreasing particle size.1
In terms of magnetic properties, the nanostructures of bulk magnetic materials can behave as soft or hard magnets with improved properties, even exhibiting super-paramagnetism at critical grain sizes.2 Non-magnetic bulk materials can become magnetic at the nanoscale, with properties influenced by composition and synthesis method.1,2
The size of nanomaterials governs their optical properties, such as reflectance and scattering. For instance, reflectance increases with particle size and decreases with increasing refractive index.2 This size-dependent optical behavior is evident in gold nanoparticles, which appear yellow at 100 nm, greenish-yellow at 50 nm, and red at 25 nm.1
Nanomaterials can increase electric conductivity in ceramics and resistance in metals. This is attributed to the quantum effects leading to electron delocalization in discrete energy states in nanomaterials. In addition, nanomaterials are great catalysts for various processes owing to their high reactivity and selectivity.1
Production Techniques
Nanomaterials are synthesized through bottom-up or top-down methods. Bottom-up methods involve building nanoparticles from atomic levels and include techniques such as sol-gel, sedimentation, reduction, green synthesis, biochemical synthesis, spinning, pyrolysis, atomic layer deposition, molecular self-assembly, and chemical vapor deposition (CVD).1,2
Top-down methods, which involve trimming bulk materials to the nanoscale, include mechanical milling, lithography, sputtering, thermal decomposition, etching, physical vapor deposition, and arc discharge.1,2
Mechanical or ball milling is the most widely used method for producing nano-coatings, metallic nanoalloys, and other nanocomposites by grinding the bulk materials to nanosize.1 Another method used in industrial applications is sputtering, which produces high-quality nanomaterials in a vacuum by depositing a thin layer of nanoparticles on the desired substrate from a bulk target.1
Nanolithography is an advanced method that uses concentrated electrons or light beams to create nanostructures on a light-sensitive material. It can produce nanostructures with specific shapes and sizes using a single nanoparticle. However, it is an expensive method requiring sophisticated equipment. Thermal decomposition, on the other hand, is a cost-effective method that uses heat to break down materials into nanoparticles.1
The sol-gel method is widely employed to fabricate metallic nanoparticles from oxide or chloride precursor solutions. Alternatively, CVD uses gaseous reactants to fabricate nanostructures on a substrate. While CVD is rapid and scalable, it requires special equipment, and the gaseous by-products are highly toxic.1 Thus, industries commonly use pyrolysis for the large-scale production of nanoparticles as it is simple, efficient, cost-effective, and a continuous process with high yield.1
Challenges and Opportunities
Nanomaterials are increasingly integral to all aspects of human life. However, their production and application face numerous challenges. For instance, defects in nanomaterials can negatively impact their intrinsic characteristics, compromising target functionality.3
Despite technological advancements, producing high-quality nanomaterials in a cost-effective and controlled manner remains challenging. High-quality nanomaterials require advanced equipment and a regulated environment, hindering scalable production. Conversely, widely employed low-cost methods for large-scale production often yield poor-quality nanomaterials with defects.3
During application, the tendency of nanoparticles to agglomerate significantly damages their performance. Severe agglomeration compromises the high surface areas and unique properties of nanomaterials.3
Another significant challenge is the toxicity of nanomaterials, which is not yet fully understood and depends on factors such as composition, size, surface chemistry, reactivity, and fabrication method. The toxicology of nanomaterials can severely impact human body processes and ecosystems through bioaccumulation.2,3
Despite these limitations, nanomaterials offer several opportunities for development. For instance, they can accelerate the diagnosis and control of diseases like COVID-19 and monkeypox. Magnetic nanomaterials are promising for biomedical applications, including drug delivery and magnetic resonance imaging.1
Nanomaterials are also promising for future technologies in clean energy production, nanomedicine, tissue engineering, energy storage, memory devices, optoelectronics, air purification, waste management, and advanced scientific tools.1-3
Emerging Trends
Continuous research and development are expanding the application scope of nanomaterials by overcoming existing challenges. For example, a recent study in Fuel demonstrated the application of nanomaterials to enhance the production of biofuels such as biodiesel, biogas, biohydrogen, and bioethanol. Using appropriate nanomaterials improves feedstock conversion rate, efficiency of separation and purification processes, and product quality.4
Another recent study in Global Challenges proposed harnessing green synthesized nanomaterials for sustainable crop production. Green synthesis can address the toxicity and cost concerns associated with chemically synthesized nanomaterials. These eco-friendly nanomaterials can be used in agriculture as fertilizers, insecticides, pesticides, and fungicides, offering economic and social benefits by enhancing agricultural productivity while minimizing environmental impact.5
In conclusion, the interaction of nanomaterials with various synthetic and biological systems depends on their physicochemical attributes, which can be optimized by improving the synthesis methods. Eco-friendly nanomaterials with no toxicity present numerous opportunities to enhance human well-being through advanced technologies and a healthy environment.
More from AZoM: What are Multiferroics?
References and Further Reading
1. Mekuye, B., Abera, B. (2023). Nanomaterials: An overview of synthesis, classification, characterization, and applications. Nano Select. doi.org/10.1002/nano.202300038
2. Saleh, TA. (2020). Nanomaterials: Classification, properties, and environmental toxicities. Environmental Technology & Innovation. doi.org/10.1016/j.eti.2020.101067
3. Baig, N., Kammakakam, I., Falath, W. (2021). Nanomaterials: A Review of Synthesis Methods, Properties, Recent Progress, and Challenges. Materials Advances. doi.org/10.1039/d0ma00807a
4. Awogbemi, O., Kallon, V. (2024). Recent advances in the application of nanomaterials for improved biodiesel, biogas, biohydrogen, and bioethanol production. Fuel. https://doi.org/10.1016/j.fuel.2023.130261
5. Sundararajan, N., et al. (2023). Mitigating Global Challenges: Harnessing Green Synthesized Nanomaterials for Sustainable Crop Production Systems. Global Challenges. doi.org/10.1002/gch2.202300187
Disclaimer: The views expressed here are those of the author expressed in their private capacity and do not necessarily represent the views of AZoM.com Limited T/A AZoNetwork the owner and operator of this website. This disclaimer forms part of the Terms and conditions of use of this website.