The structure of chemical compounds can be accurately determined using NMR spectroscopy. With NMR spectra, information about the functional groups as well as atom positions in the molecule can be obtained. This article discusses an example in which NMR can be used for determining the structure of a range of compounds all with the same chemical formula C4H8O2.
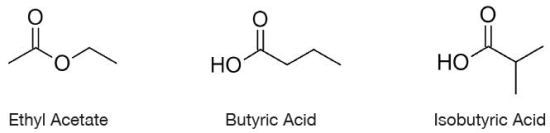
Figure 1. Chemical Structure of butyric acid, isobutyric acid and ethyl acetate
Student Instructions
The three isomers, with the molecular formula C4H8O2, comprise of varied carbon skeletons and functional groups. The three compounds are liquids at ambient temperature.
The experimental procedure involves the following steps:
- Single-dimension proton spectra of neat samples on the Spinsolve® Proton NMR spectrometer are determined
- Sample preparation is performed by pipetting around 700µL of liquid into a 5mm NMR tube
- The compound is identified for every single-dimensional proton spectrum and the resonances in the spectrum are assigned to the structure
- A table is then created for each unknown compound having five columns: chemical shift, integration value, multiplicity (splitting pattern of the peak), coupling constant and possible environment
- Chemical shift tables such as the one, shown in Figure 2 displays the normal range for chemical shifts of typical functional groups in organic compounds. When it is used along with integration values and the splitting pattern, the compound structure can be determined based on the chemical shift
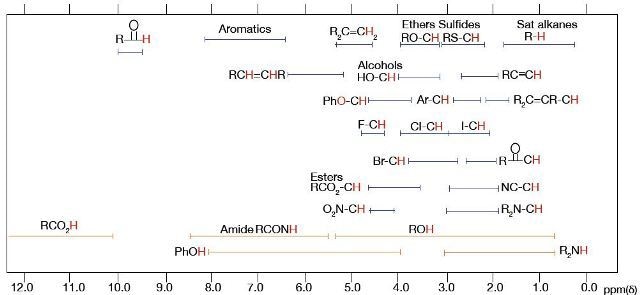
Figure 2. Chemical Shift Table
Worked Example - Compound 1
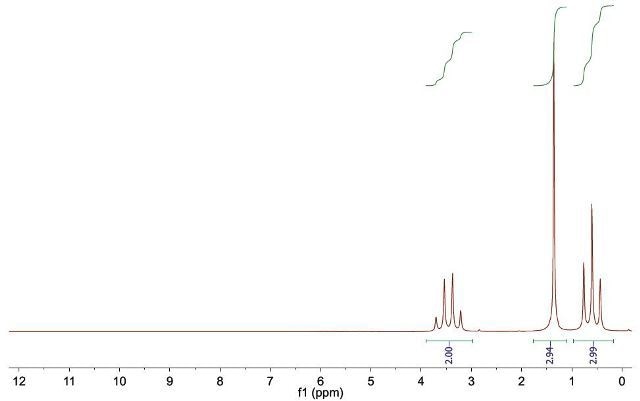
Figure 3.
Table 1. Chemical shift table for compound 1
δ 1H |
Integration |
Multiplicity |
Coupling Constant |
Environment |
3.45 |
2 |
Quartet |
7.1 Hz |
ROH, RCO2CHR, PhOCHR2, R2CHOH |
1.36 |
3 |
Singlet |
- |
ROH, R(CO)CHR2, R2C=CRCHR2,RC≡CH |
0.60 |
3 |
Triplet |
7.1 Hz |
ROH, R3CH |
It is important to note that certain of these chemical shifts and functional groups overlap each other, including atoms other than hydrogen, oxygen and carbon. It is beneficial to omit these since it has a known chemical formula and none of those other atoms are present.
The compound includes three distinct proton environments but from the molecular formula it is obvious that there are four carbon atoms. This implies that either two carbon atoms are equivalent or one carbon atom is non-protonated.
The number of protons in the single-dimensional proton spectrum comes to 8, considering all the hydrogen atoms in the molecule. At δH3.45, the chemical shift of the resonance is significantly downshield, and therefore, deshielded. Hence, it can be inferred that the carbon at this point has a single bond to an oxygen atom.
At δH1.36, the resonance is downfield to a very small extent, and its chemical shift shows that it may be a carbon-carbon or carbonyl multiple bond. It can be inferred that there must be a carbonyl in Compound 1 as none of the compounds have carbon-carbon multiple bonds. The chemical shift anticipated for a regular carbon in a saturated environment is SH 0.60.
Studying the coupling pattern of the spectrum peaks allow the assembly of building blocks for explaining the structure. Since, the proton at δH 3.45 is a quartet, it combines with three equivalent protons on the adjacent carbon atom. So, the neighboring group is a methyl. As it combines for two protons, this resonance can be considered as a CH2.
Therefore, the carbon is bonded to two hydrogen atoms and a methyl group, thus accounting for three bonds. Since additional coupling is not seen, it can be implied that the fourth bond is to a non-protonated atom. It can be seen from the chemical shift that it is an oxygen atom. Since they combine for three protons, both the remaining resonances are methyl groups.
The proton δH 0.60 is seen as a triplet in the spectrum, implying that it is a neighbor to a carbon having two equivalent protons. Hence, δH 0.60 bonds with the protons at δH 3.45 so that they are linked. The δH 0.60 is chemical shift is in agreement with this position (shielded, saturated carbon). The δH 1.36 methyl group does not have any very close neighbors as it is a singlet.
It can thus be inferred that it is not close to a protonated atom, and from the chemical shift it is adjacent to the carbonyl. By summing up the building blocks and taking into consideration the functionality suggested by the molecular formula and chemical shifts, it is inferred that Compound 1 is ethyl acetate.
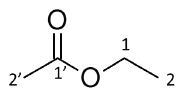
1H NMR (42.5 MHz, neat) δ 3.45 (q, J = 7.1, 2 H, H-1), 1.36 (s, 3 H, H-2’), 0.60 (t, J = 7.1, 3 H, H-2).
Figure 4. Compound 1 is ethyl acetate
Worked Example - Compound 2
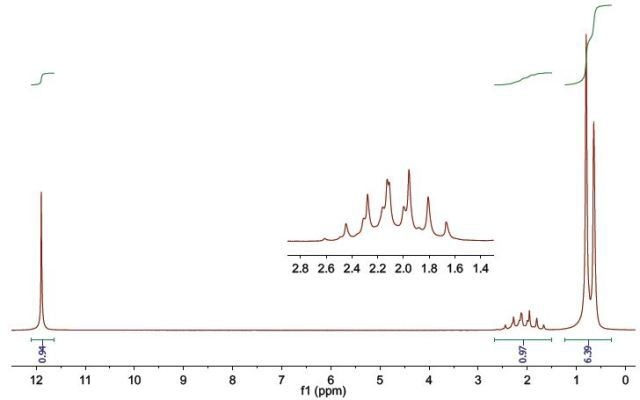
Figure 5.
Table 2. Chemical shift table for compound 2
δ 1H |
Integration |
Multiplicity |
Coupling Constant |
Environment |
11.90 |
1 |
Singlet |
- |
RC(O)OH |
2.13 |
1 |
Septet |
6.8 Hz |
ROH, R(CO)CH3, R2C=CRCHR2,RC≡CH |
0.73 |
6 |
Doublet |
6.7 Hz |
ROH, R3CH |
The number of proton environments in this compound are three and it can be observed from the molecular formula that the number of carbon atoms are four. Hence, it can be inferred that there are either two equivalent carbon atoms or one non-protonated carbon atom.
The chemical shift of the resonance at δH 11.90 has moved downfield, hence, it must be a carboxylic acid’s hydroxyl proton. This takes into consideration a hydrogen atom and two oxygen atoms. At δH 2.13, the resonance combines for a single proton, hence, it is a CH group. The small downfield shift of the resonance from the normal aliphatic protons at δH 0.73 shows that it is close to the carboxylic acid functional group.
The resonance splits at δH 2.13, giving rise to a septet. This implies that it bonds to six equivalent protons, which are at δH 0.73. The δH0.73 peak combines for six protons and is observed to be a doublet implying that it bonds with the proton at δH 2.13. There are now 2 carbon atoms and 6 hydrogen atoms, with a CH group and a carboxylic acid functional group taken into consideration. Hence, it is inferred that equivalent methyl groups are the six protons at δH 0.73.
Combining the building blocks and taking into account the functionality suggested by the molecular formula and the chemical shifts, Compound 2 is found to be isobutyric acid.
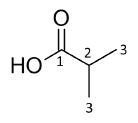
1H NMR (42.5 MHz, neat) δ 11.90 (s, 1 H, OH), 2.13 (sept, J = 6.8, 1 H, H-2), 0.73 (d, J = 6.7, 6 H, H-3).
Figure 6. Compound 2 – isobutyric acid
Worked Example - Compound 3
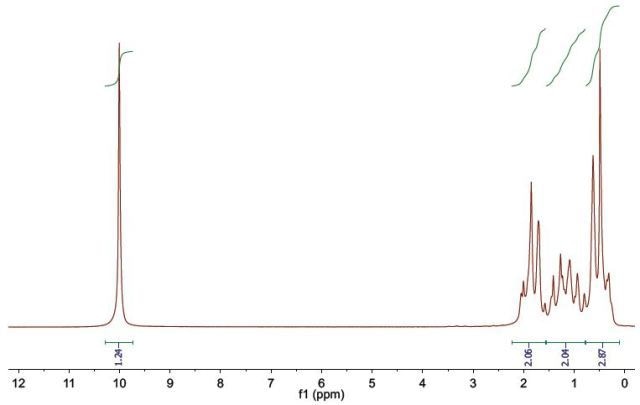
Figure 7.
Table 3. Chemical shift table for compound 3
δ 1H |
Integration |
Multiplicity |
Coupling Constant |
Environment |
10.02 |
1 |
Singlet |
- |
RC(O)OH |
2.1-1.6 |
2 |
Multiplet |
- |
ROH, R(CO)CH3, R2C=CRCHR2,RC≡CH, R3CH |
1.6-0.8 |
2 |
Multiplet |
- |
ROH, R2C=CRCHR2,RCECH, R3CH |
0.8-0.2 |
3 |
Multiplet |
- |
R3CH |
The spectrum includes four separate proton environments. The chemical shift of the resonance at δH10.02 is significantly shifted downfield, and hence, is in the chemical shift range for a carboxylic acid’s hydroxyl proton.
The three proton environments that are left are not resolved appropriately, and hence, it is tough to ascertain their multiplicity and chemical shift. This does not provide data about the structure, where these protons are in a similar chemical environment, as characterized for alkyl chains.
The peak position can be determined and combined and from these combination values,
CH2, CH2, and CH3 are observed. The distance from the carbonyl group can also be ascertained from the chemical shift range. It is, hence, observed that the CH2 group at δH 2.1 – 1.6 is the closest and CH3 group at δH 0.8 to 0.2 being the further-most. From a carboxylic acid having a 3-carbon alkyl, Compound 3 is assigned as butyric acid.
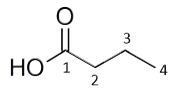
1H NMR (42.5 MHz, neat) δ 10.02 (s, 1 H, OH), 2.1-1.6 (m, 2 H, H-2), 1.6-0.8 (m, 2 H, H-3), 0.8-0.2 (m, 3 H, H-4).
Figure 8. Compound 3 – butyric acid
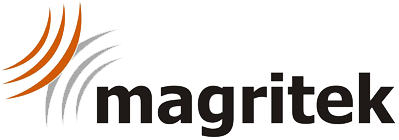
This information has been sourced, reviewed and adapted from materials provided by Magritek.
For more information on this source, please visit Magritek.