Fluorescence from particulates (e.g. Pd nanoparticles) or molecular fluorophores in the reaction mixture are a barrier to achieving Raman spectra in reaction monitoring. The presence of chromophores is another challenge.
Even when the concentration of chromophores are at submillimolar levels, their Raman scattering is capable of dominating a spectrum. One approach to overcome this issue is to move away from the fluorophores’ resonance absorption regions, and into the near infrared (NIR) spectral region.
However, when employing conventional silicon-based CCD cameras, the excitation wavelength is limited to 785 - 850 nm. The reason for this limitation is a drop in sensitivity by approximately 1 µm.
The new InGaAs based multichannel array detectors can provide a solution to this problem. The detectors can extend the sensitivities into the NIR over 2 µm, enabling excitation at longer wavelengths such as 1064 nm.
Benefits of InGaAs-Based Multichannel Array Detectors
Generally only Fourier Transform (FT)-Raman systems have been used to achieve Raman spectra with 1064 nm excitation. However, these systems are bulky and pose issues when applications require portability.
In addition, the intensity of required excitations often damages the samples when acquiring proper spectra. This creates serious issues in areas of research such as the micro-spectroscopic analysis of living cells.
With the availability of InGaAs-based multichannel NIR sensitive thermoelectric (TE) cooled detectors and compact, high quality continuous wave 1064 nm lasers, new avenues have opened up in NIR Raman spectroscopy.
This article outlines a series of illustrative spectra that were obtained using an Andor InGaAs camera (DU490A-1.7). The effect of using excitation at 1064 nm to reduce the influence of fluorescence is demonstrated in Figure 1.
The spectrum obtained from a 9-methylanthracene specimen at a wavelength excitation of 785 nm can be seen in Figure 1(A). A spectrum obtained with excitation at 1064 nm, without any post processing, can be seen in Figure 1(B). There is a visible reduction in the background fluorescence.
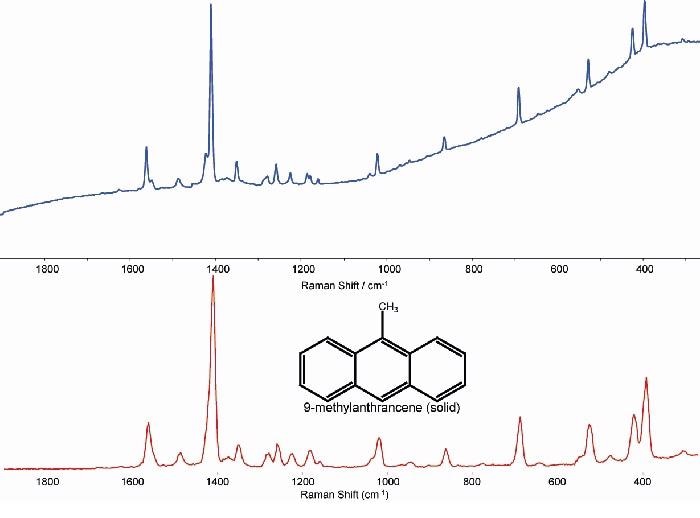
Figure 1. Spectra from 9-methylanthracene, A - ?exc of 785 nm and B - ?exc of 1064 nm.
Dispersive NIR Spectroscopy System
The dispersive NIR spectroscopy system employed in the experiment was designed around the iDus InGaAs (DU490A-1.7) photodiode array (PDA). A lens-based flange was used to connect the PDA to the Shamrock 163 spectrograph equipped with a 300 l/mm grating blazed at 1250 nm, to achieve increased signal collection (SR1-ASZ-8044).
A commercial Raman probe (Inphotonics) was employed to collect Raman scattering, with the excitation set at 1064 nm. Through a fiber, the excitation was fused with a CW 1064 nm laser that was applied to the specimen at 200 mW power.
A ‘circular to line’ short fiber optic was used to feed the Raman signal into the spectrograph. The schematic of the system used is demonstrated in Figure 2.
Figure 3 shows the iDus InGaAs spectrograph and camera. The camera resolution is set at 12 cm-1 and measured using calcite.
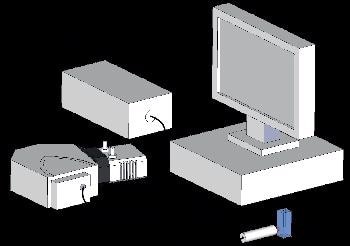
Figure 2. Schematic of experimental setup showing the Raman probe delivering the excitation source to the sample, collecting the Raman signal and delivering it into the spectrograph.
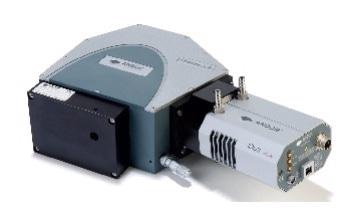
Figure 3. The iDus InGaAs on a Shamrock 163 spectrograph.
Figure 4 demonstrates a few sample NIR Raman spectra. The general parameters used include exposures of 5 seconds with 4 accumulations, while the slit width of the spectrograph was set at 10 µm. The spectra were background corrected without the need for postprocessing.
2 solid sample, 20 mW at sample, sum of ten 5 s exposures; (iii) [Mn2O3 (trimethyltriazacyclon onane)2](PF6)2 solid sample, 20 mW at sample, sum of twenty 10 seconds exposures. Spectra are background corrected. No post acquisition processing has been applied.](https://d12oja0ew7x0i8.cloudfront.net/images/Article_Images/ImageForArticle_13137_4432343166063668557.jpg)
Figure 4. Sample Raman spectra taken in the NIR with a CW Nd-YAG laser at 1064 nm and the iDus InGaAs on a range of different organic and inorganic solid materials. (i) Acetonitrile/toluene (1/1 v/v), 200 mW laser power at sample, sum of five 4 s exposures; (ii) [Ru(bipy)3](PF6)2 solid sample, 20 mW at sample, sum of ten 5 s exposures; (iii) [Mn2O3 (trimethyltriazacyclon onane)2](PF6)2 solid sample, 20 mW at sample, sum of twenty 10 seconds exposures. Spectra are background corrected. No post acquisition processing has been applied.
The quantum efficiency (QE) of two InGaAs detectors that has extended sensitivities of 1.7 µm and 2.2 µm, respectively, can be seen in Figure 5.
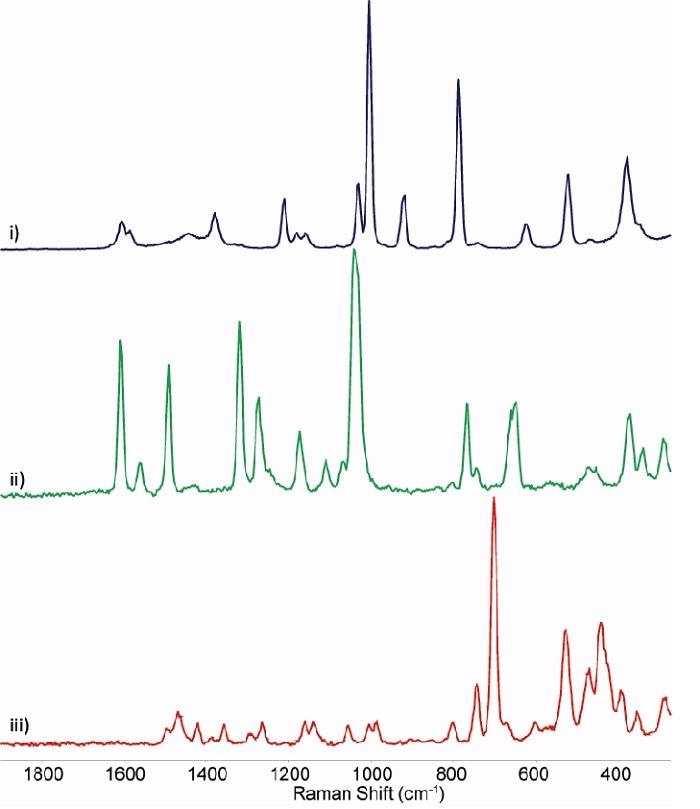
Figure 5. Quantum Efficiency (QE) characteristics of two different InGaAs detectors with extended sensitivities to 1.7 µm and 2.2 µm respectively.
There is one major issue with the NIR Raman. Specimen heating in the NIR creates a significant amount of background emission, particularly with 1064 nm Raman.
Even solid samples that do not absorb light at this wavelength can heat up quickly. Therefore, the detection system must be sensitive enough to enable low laser sensitivities at the specimen. The examples in Figure 5 (ii and iii) show immediate specimen burning at 200 mW laser power.
Sample heating can be prevented by reducing the power to a mere 20 mW, while still obtaining a signal-to-noise ratio (S/N) spectra within a period of less than 4 minutes.
Conclusion
InGaAs detectors with different extended sensitivities into the IR are available, however the materials required to extend sensitivity further into the IR tend to have significantly higher levels of dark noises.
Cooling the InGaAs sensor in the iDus can be reduced to -90°C due to thermoelectric (TE) cooling. At this temperature, the intrinsic sensor noise is significantly reduced below that due to the ambient background.
The TE cooling is both convenient and reduces running costs. In addition, when compared to conventional liquid nitrogen (LN2), TE cooling is easy to implement in portable systems.

This information has been sourced, reviewed and adapted from materials provided by Andor Technology Ltd.
For more information on this source, please visit Andor Technology Ltd.