Carbon and oxygen have a high absorption contrast. This is used to study biological and mineral samples via the “water window“, which has a spectral range between λ = 2.3 – 4.4 nm.
Transmission X-ray microscopy in this range is of immense use, when Fresnel zone plates are used as objective lenses with their high magnifications, because it is possible to obtain spatial resolutions at 10 nm. The limitation is the need for a synchroton source to provide photons of high frequency at a sufficiently high flux for such experiments to be carried out.
Other sources which use gas discharge or laser-produced plasmas have been explored as laboratory sources of photons, so that soft X-ray microscopy can be routinely performed without the need to wait on beams at large-scale facilities to become free.
Plasmas produced by lasers are especially successful and have been employed in applications belonging to various fields, from material ablation and structuring to absorption spectroscopy and transmission X-ray microscopy.
In this application note, a soft X-ray microscope of unusual compactness is described. It has the operational range of the “water window” and uses Helike nitrogen-produced radiation of a single wavelength at 2.88 nm. The source is laser-induced plasma from a pulsed jet of gas, with almost zero debris and long-term stability.
Experimental Setup
Figure 1 shows the setup of the soft X-ray microscope, a tabletop device with a plasma source which is laser-induced, an ellipsoidal condenser mirror, an objective composed of a Fresnel zone plate, and a CCD camera with back illumination which responds to soft X-rays.
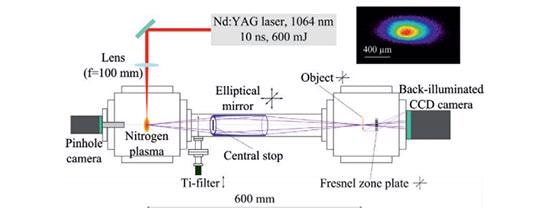
Figure 1. Schematic drawing of the table-top soft X-ray microscope. The inset shows a pinhole camera image of the laser-induced nitrogen plasma averaged over 10 pulses recorded for the emission wavelength of 2.88 nm
Soft X-rays are quickly absorbed by air, and therefore the current set of experiments was carried out in a vacuum system at a base pressure of 1*10-6 mbar. The plasma source uses an Nd:YAG laser system (Quantel) based on a gas target, and has a wavelength of 1064 nm and pulse energy of 600 mJ, with a pulse duration of 10 ns and repetition rate of 5 Hz.
The nitrogen plasma emits rays that pass through a Titanium filter 200 nm thick, which blocks radiation of other bandwidths including visible light spectrum and scattered laser radiation. Radiation of λ = 2.7 nm falling at the L-edge of the titanium plate and below it is also absorbed so that the samples receive purely monochromatic radiation of λ = 2.88 nm.
A pinhole camera with hole diameter of 50 microns is used to monitor the spatial distribution of the plasma. The size of the nitrogen plasma at this wavelength is 0.44*0.24 mm² (FWHM) by measurement.
After irradiation of the sample, the soft X-rays are collected and focused using an axisymmetric ellipsoidal condenser mirror with a nickel coating from Rigaku, Inc. It has a focal length of 300 mm and a mirror length of 100 mm. The object plane receives the focused radiation from the sample, which is then imaged using a Fresnel zone plate objective (ZonePlates Ltd.) of 190 microns, a minimum zone width drn of 30 nm, and with 1580 zones.
The image falls at up to 500 times magnification on to a CCD camera (Andor iKon-L SO model DO936NOW-BN) with back-illumination and a pixel size of 13.5*13.5 square microns, or 2048*2048 pixels) which is soft X-ray sensitive.
Results and Discussion
At first, the soft X-ray microscope was tested for imaging over its whole field of view (FOV), approximately 50 microns, by recording a Siemens star test pattern (NTTAT, model ATN/XRESO-50). As seen in Figure 2, the full FOV showed near-uniform illumination on the resulting micrograph. The inset shows that good resolution is available in all directions with the smallest (50 nm) Siemens star structures.
Next, as seen in Figure 3, Deinococcus radiodurans, which is a polyextremophilic bacterium, was subjected to imaging, which showed its representative structure with clarity. This was followed by test imaging of other microbes enriched with iron, due to which they absorbed radiation better than corresponding carbonaceous material.
The experiment also included examination of geocolloids segregated from a central German river, the Main, which depicted both irregular and microstructured particles. The lower part of the figure shows a structure resembling a necklace which is attributed to biological debris. The holes are isolated and regularly spaced at about 215 nm which demonstrates the instrument’s excellent resolution.
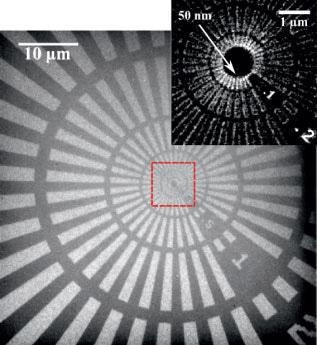
Figure 2. Soft X-ray micrograph of Siemens star recorded at λ = 2.88 nm (magnification 250x, effective pixel size 52 nm, 18000 pulses, exposure time 60 min). The inset shows the central part of the Siemens star recorded separately at magn. 500x.
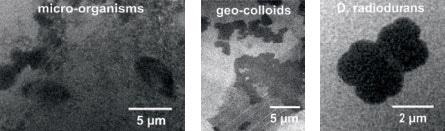
Figure 3. Soft X-ray micrograph of (left) bacterium Deinococcus radiodurans, (middle/below) iron enriched micro-organisms and (right) geo-colloids taken from the river Main recorded at λ = 2.88 nm (magnification 250x, effective pixel size 52 nm, 18 000 pulses, exposure 60 min). The D. radiodurans was provided by T. Salditt (University of Göttingen), the micro-organisms and geocolloids were collected by J. Niemeyer (University of Göttingen).
Conclusion and Outlook
The experimental setup showed that a tabletop soft X-ray microscope with an operational wavelength of 2.88 nm is feasible, being based on laser plasma from a gas target, which produces practically no debris and is stable over the long term. At present it is capable of detecting structures 50 nm or more in size, though the plasma brilliance is comparatively low which offers a low ratio of signal to noise.
However, it is possible to scale the photon flux, a phenomenon which has been independently studied already. As a first step, a laser with higher average power (or repetition rate), or which has shorter pulses, or both, could be substituted. When isoenergetic ps laser pulses are used instead of ns pulses, as at present, this will produce greater excitation and hence brighter plasma by over 10 times, within the “water window” range.
If particle densities are higher, as may be achieved by increasing the gas pressures or using a barrel shock technique, the plasma may become at least one order of magnitude brighter. Such modifications will help make the system more sensitive, reducing the period of exposure to take a micrograph. This keeps the system size small and preserves the inbuilt cleanliness of the source of the soft X-rays.
References and Further Reading
- W. Meyer-Ilse, D. Hamamoto, A. Nair, S. A. Lelièvre, G. Denbeaux, L. Johnson, A. L. Pearson, D. Yager, M. A. Legros, and C. A. Larabell, J. Microsc. 201, 395–403 (2001).
- M. Juenger, V. Lamour, P. Monteiro, E. Gartner, and G. Denbeaux, J. Mater. Sci. Lett. 22, 1335-1337 (2003).
- W. Chao, P. Fischer, T. Tyliszczak, S. Rekawa, E. Anderson, and P. Naulleau, Opt. Express 20, 9777–9783 (2012).
- M. Benk, K. Bergmann, D. Schäfer, and T. Wilhein, Opt. Lett. 33, 2359-2361 (2008).
- M. Berglund, L. Rymell, M. Peuker, T. Wilhein, and H. Hertz, J. Microsc. 197, 268-273 (2000).
- F. Barkusky, C. Peth, A. Bayer, and K. Mann, J. Appl. Phys. 101, 124908 (2007).
- Bartnik, H. Fiedorowicz, R. Jarocki, J. Kostecki, A. Szczurek, and M. Szczurek, Appl. Phys. B 96, 727-730 (2009).
- Peth, F. Barkusky, and K. Mann, J. Phys. D: Appl. Phys. 41, 105202-105207 (2008).
- M. Müller, T. Mey, J. Niemeyer, and K. Mann, Opt. Express 22, 23489-23495 (2014).
- P. Wachulak, A. Torrisi, A. Bartnik, D. Adjei, J. Kostecki, L. Wegrzynski, R. Jarocki, M. Szczurek, H. Fiedorowicz, Appl. Phys. B 118, 573-578 (2015).
- M. Müller, T. Mey, J. Niemeyer, M. Lorenz, and K. Mann, AIP Conf. Proc. 1764, 030003 (2016).
- S. Kranzusch and K. Mann, Opt. Commun. 200, 223-230 (2001).
- M. Müller, F. Kühl, P. Großmann, P. Vrba, and K. Mann, Opt. Express 21, 12831-12842 (2013).
- T. Mey, M. Rein, P. Großmann, and K. Mann, N. J. Phys. 14, 073045 (2012).

This information has been sourced, reviewed and adapted from materials provided by Andor Technology Ltd.
For more information on this source, please visit Andor Technology Ltd.