Sponsored by HORIBAJun 12 2018
Fluorescence anisotropy is a measurement of how a molecule changes its orientation in space, with respect to the time between absorption and emission events.
Absorption and emission are the core indicators of the spatial alignment of a molecule’s dipoles, relative to the electromagnetic wave of excitation and emitted light. To look at this another way, if the fluorophore population is excited with vertically polarized light, then the emitted light will retain some of that polarization based on how fast it is rotating in solution.
The amount of depolarization of the emitted light is linked to the speed of the orientation motion – fast orientation motions will cause more depolarization and vice versa.
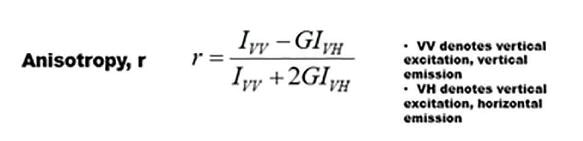
Figure 1. Anisotropy equation.
In order to make use of this information, polarizers are inserted into the excitation light path and the emission light path of a fluorometer. Anisotropy is calculated looking at the ratio of intensities in the above equation, and here IVV indicates the intensity with vertically polarized excitation and vertical polarization on the detected emission.
IVH highlights the intensity when using a vertical polarizer on the excitation and horizontal polarizer on the emission, while G is a grating factor used as a correction for the instrument’s differential transmission of the two orthogonal vector orientations.
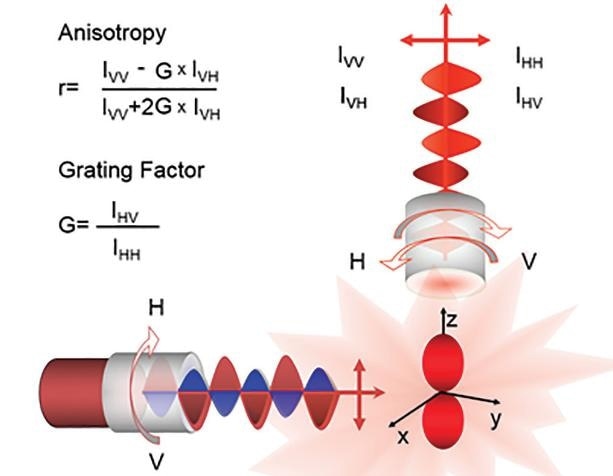
Figure 2. Depiction of fluorescence anisotropy experiment using excitation and emission polarizers rotated to 0 degrees (Vertical, V) and 90 degrees (Horizontal, H) orientations.
This experiment works by first measuring the fluorescence using the both excitation polarizer and emission polarizer set at vertical. The intensity is then entered into the anisotropy equation as IVV, before the measurement is repeated with the emission polarizer then set at horizontal orientation; the intensity for this is entered into the equation as IVH.
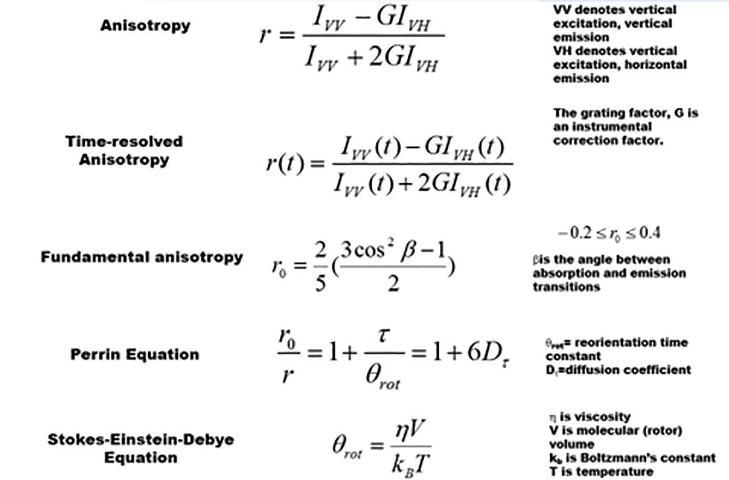
Figure 3. Some useful equations for applying anisotropy, r and time-resolved anisotropy, r(t). (Lakowicz, 2006) (Valeur, 2002)
This formula contains a factor of two because there are two orthogonal orientations that the deflection from the VVs vector can be projected onto – these being Hx and Hy, resulting in two IVH components.
Degree of polarization p is often used to illustrate a two dimensional polarization parameter where only one horizontal component is accounted for. In this case the formula would not contain the multiplier 2 for IVH where p takes the place of r.
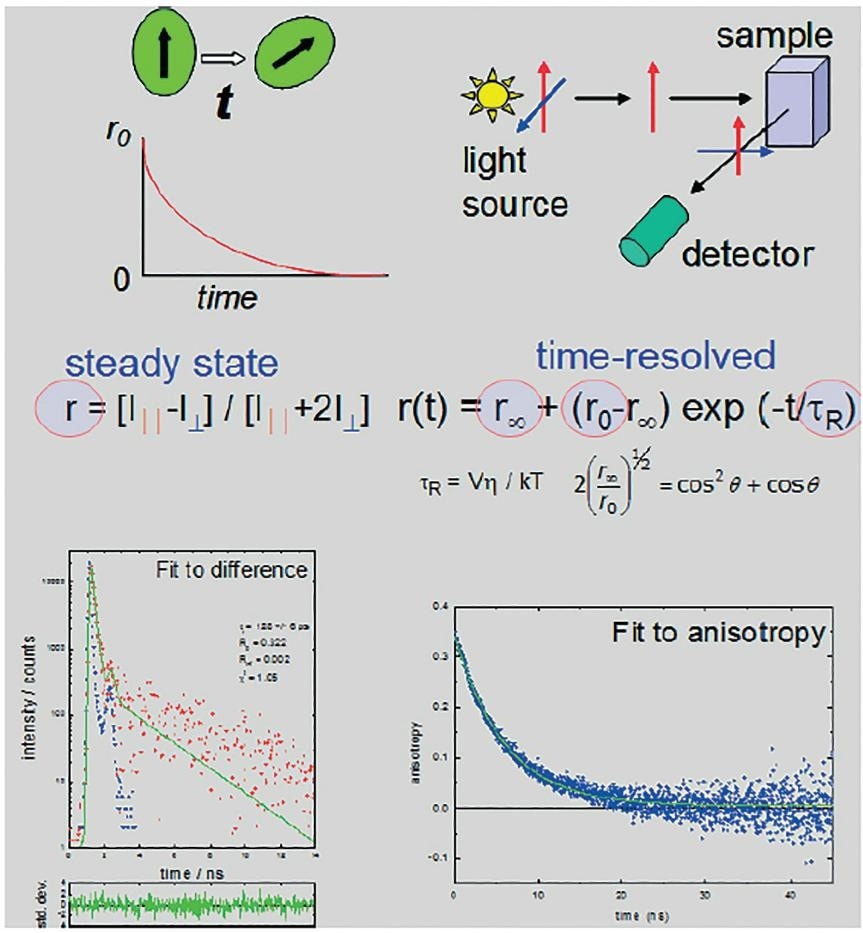
Figure 4. Time-resolved anisotropy measurement
Additionally, the G-factor is calculated by measuring the intensity at HH and HV, then inserting IHV and IHH into the equation for G. Anisotropy, shown here as a lower case r is frequently used as an indicator of molecular diffusion, size, and viscosity.
What are the Applications of Fluorescence Anisotropy?
Below it is possible to see a series of equations which can be used for the analysis of anisotropy results. The basic anisotropy equation has already been outlined above, but this can also be calculated for whole fluorescence decays, allowing for the calculation of time-resolved anisotropy.
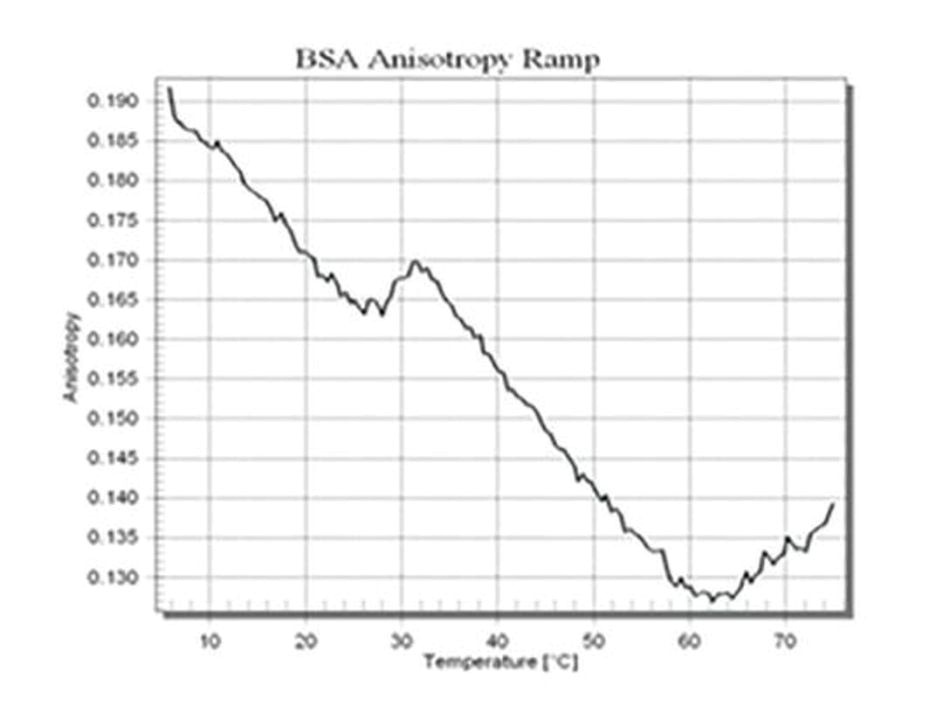
Figure 5. Temperature induced unfolding of BSA protein monitored by fluorescence anisotropy of intrinsic tryptophan residues
Once time-resolved anisotropy has been calculated, it is possible to obtain reorientation time constants and then invoke the Perrin equation and Stokes Einstein Debye equation to gain approximate values for properties like local viscosity, diffusion coefficient and molecular volumes.
These properties relate to crucial information when examining applications like polymer aggregation, protein or molecular binding; or a range of other local environment studies using complex materials and solutions.
In the previous example it is possible to see a temperature dependent protein unfolding behavior of BSA which has been measured by fluorescence anisotropy. In this case, the anisotropy of the intrinsic tryptophan residues has been used.
What are the Uses of Fluorescence Kinetics?
Fluorescence kinetics involves the observation of fluorescence intensity over time. This means, in practice, that a sample is excited at a single wavelength while the emission is detected at a single wavelength over time. Occasionally, wavelength pairs may be used for ratiometric dyes or to record baseline or background information at the same time.
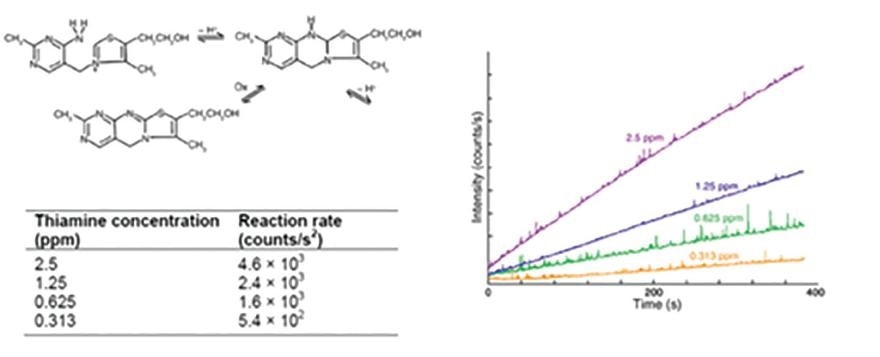
Figure 6. Top left: Reaction of thiamine and Hg2+ to form thiachrome. Bottom left: Reaction rates for thiamine standards. Right: Plots of fluorescence intensity versus time for conversion of thiamine to thiachrome for four thiamine standards. Linearity indicates a constant reaction rate for each standard.
The illustration below shows how reaction rates can be followed by using time-based measurements. In this particular example, the reaction rates of the binding of thiamine and mercury to form thiachrome are discovered by changing the concentration of thiamine used. Each individual kinetics scan here signifies a different reaction rate of the thiachrome formation reaction.
A fast mixing accessory known as a stopped flow is generally employed for the measurement of fluorescence kinetics. A stopped flow is able to mix two or more solutions together in just a few milliseconds, meaning that reaction or binding can be observed and recorded closer to mixing time zero without any diffusion effects.
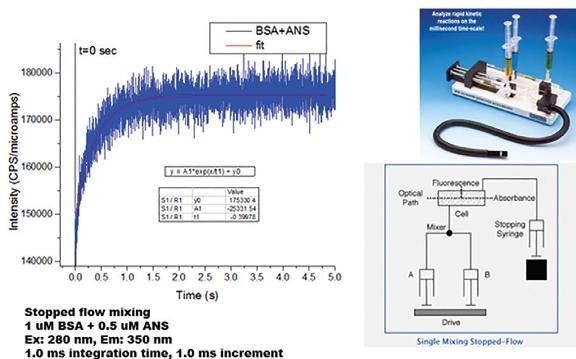
Figure 7. Left: ANS fluorescence intensity vs. time for a stopped flow mixing of BSA and ANS to measure ANS binding to the protein measured on a HORIBA FluoroMax-4. Top Right: Stopped flow fast mixing accessory. Bottom right: Schematic of the stopped flow accessory.
The image above shows the binding of a fluorophore called ANS to a protein. As can be seen here, ANS fluorescence increases on binding, so fluorescence kinetics can be used to measure the rate of binding in this instance. In this case, the binding of ANS to BSA happens with a rate of approximately 400 milliseconds.
How Can I Control the Sample Temperature on a Fluorometer?
Two very useful accessories for controlling the temperature of a sample on a fluorometer are Peltier temperature controllers and circulating baths. Regular cuvette holders found on fluorometer systems possess a pair of connections that allow for liquid circulation. These can be connected to a recirculating water bath, enabling temperature regulation between -40 °C and 70 °C.
Circulating baths are useful where a temperature must be set and held during an experiment, but a Peltier temperature controller is a more appropriate choice where samples are highly sensitive to temperature changes or must be measured at different temperatures over a range.
The Peltier controlled cell holder has a much quicker response than a traditional water bath, enabling temperatures within the range of -25 °C to 105 °C to be regulated. These devices are able to achieve a much more precise level of temperature control than a circulating bath which has the tendency to ramp up to a temperature, go over it and then return to it until the target temperature is reached.
As a further option, it is possible to use cryostats and associated mounting kits. These are available for various models of liquid nitrogen and helium cryostats and besides cooling, most of these devices are also able to heat samples up to 500 K and more.

This information has been sourced, reviewed and adapted from materials provided by HORIBA.
For more information on this source, please visit HORIBA.