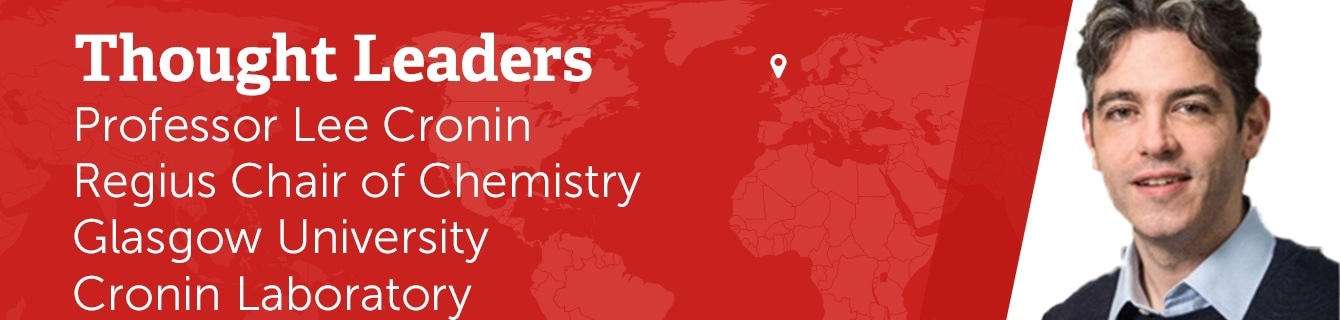
In this interview we speak to Professor Lee Cronin, regius chair of chemistry at Glasgow university about his current research and how he is using self optimising reactor systems to break new ground and open up the field of chemistry
What are you currently working on?
My research group has between 50 and 60 people in it, depending on the time of year, and we've got four big missions. The first science mission is to work out how chemistry turned into biology. The second one is to digitize chemistry, and that's discovery as well as making things. The third is to make a chemical computer. The fourth is to work out what it is about chemical systems that allow them at some point to process information.
That one sounds the most abstract because there's lots of words that maybe need an explanation, like chemistry processing information, what does that mean? Well, as opposed to messy chemistry that's complicated to understand, well the chemistry is producing data that can be read by another system. So, it's another way of asking about the origin of life.
In essence, I'm a synthetic chemist and I'm interested in how we can make systems that process information, or respond to control, to digital control, to do things by chemical synthesis or discovery.
How did you come up with this self-optimizing reactor system?
Well, what I did was to imagine the origin of life being like a chemical snowball starting at the top of a hill into an avalanche. Which meant what I wanted to do was to start a sequence of events whereby one chemical reaction would lead to another chemical reaction, which would lead to another. I imagined this as a nested flow system where I'd start doing chemistry under flow in a river or under flux, where there's chemicals being mixed in motion. The outcome of those reactions would be mixed with new chemicals and new motion, and everything would go downhill like the snowball turning into an avalanche.
So, the way I then made that in the lab was using flow systems connected together to do interesting chemistry. The initial idea then became more of a practical reality when I had to use some kind of spectroscopic assay to tell me if the compounds were interesting or not. I then segmented that project and we got to what could be described as the sensor segment, which was using the NMR.
One day, one of my post docs was saying that we need a better sensor if we're going to do the flow chemistry, "What about this NMR machine? It's quite cheap and we can dump it in the fume hood." I looked at it, and I realized that there was a hole down the middle, and even though no one had ever suggested it could be a flow NMR before, I realized I could turn it into a flow NMR, which is what I did. I think I was the first person to do that, and that's how I came up with the system.
Why is it useful that it's primarily self-optimizing?
The reason why we wanted it to be self-optimizing is we wanted it to follow reactivity, there are a number of different pieces of work we did; first of all there was the paper in the RSC (DOI: 10.1039/c4sc03075c) where we took a couple of reagents and looked at the outcome using NMR. Since we were able to see the outcome in real time, we could then go back and adjust the inputs, and look at the output immediately. A lot of what people do in chemistry is they do a reaction, they then stop the reaction, and then analyse it, and then start a new reaction with different reagents, and then look at that reaction again.
The way I look at it is this way, it's a bit like adjusting the parameters for an autopilot, where you want the plane to take off and land. But in the middle of the flight you can adjust the speed and the trajectory and get feedback from the GPS or ground radar about the location of the plane. I view the different products to be the different locations of the plane, and I wanted to move between them. I move between them by changing the flow rates or the temperature. Because the NMR is inline, I can get pretty much instant feedback about what was going on.
The first trick was realising we could do it in line, and the other trick was programming the NMR so we could get the data out fast and processed within a minute or two. The final thing was making sure we did the reactions at concentrations that the experiment could be done in real time.
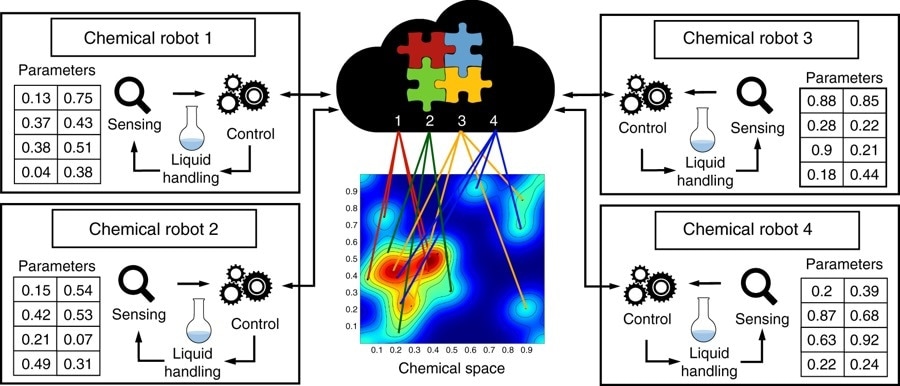
Schematic describing the concept of real-time networked chemical robots. Here four physically separated units (ChemPUs) are connected to a cloud via the internet. They receive the reactions parameters from the cloud in order to explore a chemical space in an optimised way, when the reactions are done the analysis results are returned and shared trough the cloud.
Image Credits | Dario Caramelli, Daniel Salley, Alon Henson, Gerardo Aragon Camarasa, Salah Sharabi, Graham Keenan & Leroy Cronin, https://doi.org/10.1038/s41467-018-05828-8 in accordance with the Creative Commons Attribution 4.0
What have you achieved with your system so far?
Well we haven't made a life form yet, we're working on that, but in terms of the small kind of goals and achievements, "Can we make a reaction optimizer?", well that's done but we're making it more general. Can we use it to follow reactivity? Well, the paper we published in Nature a few weeks ago was the start of that, but there's so much more to do.
A lot of organic chemists are target-led, so everyone thinks of a target then just work towards that, everyone thinks I'm a bit crazy when I say, "I don't want to have a target. I just want to follow reactivity and see where it takes me," but I think that's an entirely natural question for a scientist, for an explorer to do. To point the USS Enterprise into the middle of space and go, "That way." That's what I wanted to do I wanted to basically do Star Trek chemistry. We've started doing that and we've shown that we can discover new reactions so the goals are partly fulfilled, but there's a lot more to do.
Organic chemistry's much more important than just science, It's about making drugs, solving problems, keeping people safe, there are so many other layers there. However where I'm coming from is I'm an explorer scientist, I want to know how the world works. For me, asking the awkward question, which is just go into new chemical space and have no expectation, is entirely justified, but it's very hard for a bench organic chemist to justify. If you say to an organic chemist, "How are you going to go discover something new?" They will probably say, "Oh, I wouldn't. I'd write down a new molecule that I knew I could make," and they'll try and make it. Then you'll be like, "But if you just make what you write down, is that really interesting?"
Now of course people make mistakes because they write down what they want to make, and then they don't make that. Something else happens and then they follow that instead, and that's when they make the discovery and I think that’s a really exciting thing to happen.
The other thing is just to create reactivity where we don't currently look for or test reactivity, you should question how do you invent new reactions and how do you invent reactions that are novel? The way I would do it is I would just mix stuff together that's never been mixed together before and see where it takes me.
I would also pick materials that have an ambiguous bonding interaction, that's why samarium iodide reagents are weird, because they have different hypervalency available. What I'm doing in the next piece of work, and as a follow-up to my Nature paper, is going to different parts of the periodic table where bonding is ambiguous. I don't want a nucleophile, I don't want an electrophile, I want a system that can become electrophile one day and a nucleophile on the other depending on the circumstances. This links back to my work with digitally controllable systems and being able to change these myself.
I mean you might detect the way I do science is I just make shit up, see how many people it offends, then the more people become offended, the more I do it because it's so much fun.
I think it’s because people naturally tell you something isn't going to work or they feel because organic chemistry is not a science, it's like a bespoke black discipline. There are certain ways of doing things, and anyone who doesn't do it that way is not accepted in the community and for me, that's an easy way to disrupt science and do something new.
So what does the self-optimizing system comprise of?
The one in the paper is an NMR machine, a tube reactor, a series of inputs which are basically syringe pumps with the reagent in, and some means of heating or cooling. All of them are connected to a digital processor which looks at the NMR output. Then, depending on the number of peaks and the intensity and the relative growth, the system adjusts the parameters using a given algorithm, be it a simplex algorithm or my favoured random forest algorithm, to change the various parameters, flow rates, temperatures etc.
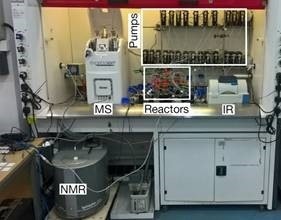
Lee's full reactor system as used in the lab with the Magritek Spinsolve system in the bottom left
Why is using a non-deuterated solvent so useful in the NMR system?
Simply put, because we can run it unlocked, it's cheap and it's just like running in a real reactor. The problem when you start to use deuterated solvents, particularly if they're not D2O, is that it becomes rapidly very expensive and therefore changes the way you do experiments, and in turn may not entirely reflect reality.
How has the benchtop size of your NMR system been beneficial for the throughput?
I don't think it's been beneficial for the throughput especially, I think it's beneficial for us designing the experiment. When I bought the NMR initially, I was like, "Does the hole go all the way through the machine? Can we then place the NMR next to a fume hood? If so, we can design a synthesis and reactivity robot around it." In effect we actually designed the robot around the machine, rather than wanting it to address a particular problem, we view the NMR as another sensor for our robot, and we designed the robot around it.
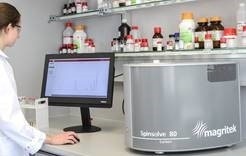
The ground-breaking Spinsolve 80 from Magritek, leading suppliers of benchtop NMRs
What would you like to see in new NMR systems?
What I would like to have is a more sensitive bench top system if possible. That means I can use lower concentrations, and therefore explore different chemical space, and more nuclei if possible, that's it.
What I need to put in a given fume hood is not just an NMR, but I want mass spec and infrared as well, or raman, because I want to give the robots the sight, sound and touch or taste. So I’d like to see developments in all the parts of the whole system that I’m using.
What are you looking to move towards off the back of your latest Nature paper?
I want to be able to use reactivity as a new way of investigation for chemical space and discovering novelty in reactions. I would love to be able to discover reactions using this approach that could not be discovered any other way, but once we've discovered them, we update the normal chemical rules which then allows us to make new molecules in a way that we understand.
So if we invent a new set of rules we may be able to make new reactions using this reactivity. We're then able to translate that and give it to bench chemists, and then they can use those rules to do reactions they couldn't do before with transformations, make bonds they couldn't make before. I guess if we do that, then the organic chemists might even accept us because then we become very useful to them.
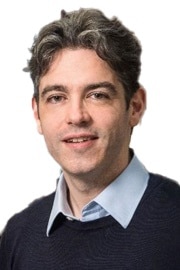
About Professor Lee Cronin
Lee Cronin was born in the UK and was fascinated with science and technology from an early age getting his first computer and chemistry set when he was 8 years old. This is when he first started thinking about programming chemistry and looking for inorganic aliens. He went to the University of York where he completed both a degree and PhD in Chemistry and then on to do post docs in Edinburgh and Germany before becoming a lecturer at the Universities of Birmingham, and then Glasgow where he has been since 2002 working up the ranks to become the Regius Professor of Chemistry in 2013 aged 39. He has one of the largest multidisciplinary chemistry-based research teams in the world, having raised over $35 M in grants and current income of $15 M. He has given around 400 international talks and has authored over 400 peer reviewed papers with recent work published in Nature, Science, and PNAS.. He and his team are trying to make artificial life forms, find alien life, explore the digitization of chemistry, understand how information can be encoded into chemicals and construct chemical computers.
Disclaimer: The views expressed here are those of the interviewee and do not necessarily represent the views of AZoM.com Limited (T/A) AZoNetwork, the owner and operator of this website. This disclaimer forms part of the Terms and Conditions of use of this website.