In the fifteen EU member states there are approximately 42,000 reported deaths and 1.5 million people injured as a result of road accidents every year. Decisions to undertake countermeasures are based on their casualty reduction potential, with identified short and medium-term priorities being to continue and increase support for Euro NCAP, and legislation on safer car fronts for pedestrians and cyclists.
Much of the technical advice on secondary vehicle safety in Europe is developed by the European Experimental Vehicles Committee (EEVC), the working group of which advises on various aspects of safety.
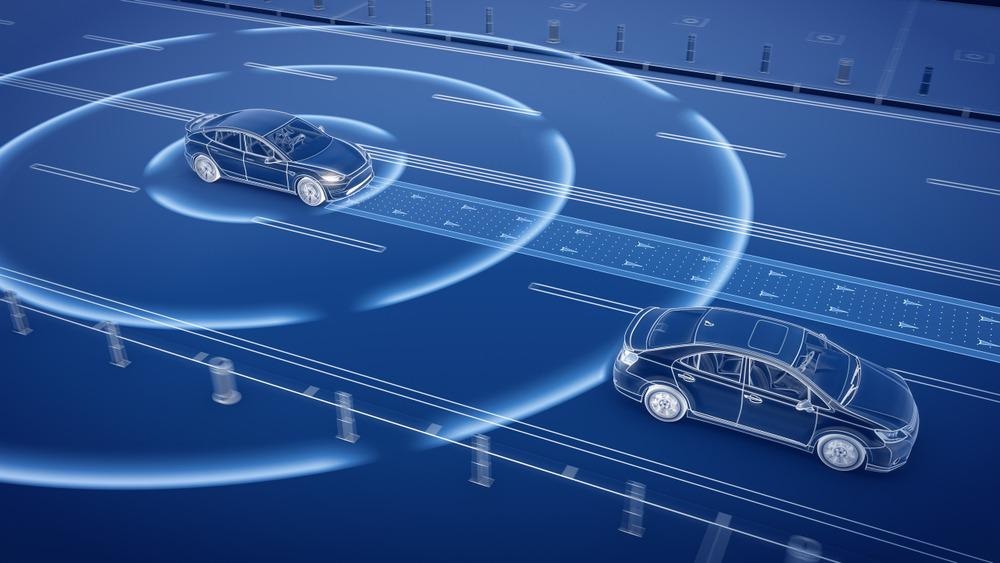
Image Credit: temp-64GTX/Shutterstock.com
Pedestrian Fatality Statistics
Among other things, it is currently looking at pedestrian protection, because in the 12 months ending June 1999, pedestrian fatalities accounted for 28% of all UK road fatalities. This compares with 13% in the USA, 28% in Japan, 18% in Australia, and 16% averaged across the rest of Western Europe. It’s a stark fact that pedestrians struck by the front of a vehicle account for 84% of pedestrian fatalities. Head injuries, most of which involved impacts with the vehicle, were the cause of death in two-thirds of the fatalities.
Testing to Reduce Pedestrian Injury
Ways to reduce this toll through improved frontal design of vehicles have been hampered by a lack of objective tests to simulate pedestrian impacts, although in the late 1980s the EEVC began to develop a set of standards that would minimise serious injury to pedestrians in impacts up to 40 km/h (25 mph), proposing a set of component tests representing the three most important mechanisms of injury - head, upper legs and lower legs of pedestrians. This was incorporated in its crash test program from 1996 with the first results published in the following year.
The Role of Materials in Safety and Design
Advanced materials have a key role to play in making cars safer for both pedestrians and passengers, by acting as energy absorbers to cushion the blow or impact in an accident. To meet the present and increasing demand for enhanced safety in cars, the ideal energy absorber should work as a multifunctional element. It should also function as a structural component, a heat exchanger, an air filter, a liquid storage component, or an acoustic insulator.
Other important considerations that must be considered are the material’s recyclability and minimisation of weight. Energy absorbing materials are seen as a way of meeting many of the challenges that are faced by designers in making cars safer, and of these, PressLoad from Cellbond Composites holds particular promise.
Impact Absorbers
The ability of the absorber to give consistent results under various impact angles, its ease of manufacture, its performance under various environmental conditions and its ease of handling are all of concern to car manufacturers. Sandwich structures can offer designers many benefits. They were traditionally made from foams (PU and PP), plastic ribs or honeycomb materials but tended to be expensive.
The PressLoad Alternative
PressLoad uses a specially designed geometry, an arrangement of regular flat-top cones, basically an egg box shape, to provide strength plus the ability to deform in a predictable way. The material consists of evenly spaced flat top cones and it is possible to tailor the shape for a given application. It is a low-cost component with excellent energy absorption capabilities and is particularly suitable for high volume production as it can be manufactured from a range of metallic sheet products (cold forming) or thermoplastic materials (thermoforming, injection moulding). The shape of the structure, whether in its raw form or sandwiched, has the additional advantage of having an open structure along its length, making it possible for gases and wiring to pass along the length of the panel.
The PressLoad Structure and its Flexibility
Figure 1, shows how the PressLoad structure is defined. The properties of the structure can be adjusted by varying the interpitch (the distance between the centre of the cones), peak diameter and cell angle for a given material choice. Reducing the interpitch has a pronounced effect on the properties of the structure this reduction gives a higher density of cones throughout the structure which in turn results in an increased stiffness and a higher plateau stress.
Behaviour in a Static Crush Test
Figure 2 shows the behaviour of PressLoad during a static crush test. The picture shows that a bending process occurs during the crush, and it is this mechanism that gives the plastic plateau, with the theoretical limit for this plateau about 65% elongation. A dynamic simulation of the PressLoad absorber demonstrates all the intrinsic qualities of this geometry, but the important features are that the material has no elastic peak, has a plastic plateau energy absorption area, and an inflation at the end of the deformation.
Under a static crush loading the walls of each PressLoad cone collapse. It is this bending of the pitch structure that is responsible for the length and homogeneity of the plateau. The parameters for adjusting the stress level include the material that the Pressload is formed from, the wall thickness of the cones, the shape of the cones, and the density of the cones. Skin could be used in order to obtain a better mechanical homogeneity in the compression direction, while a sandwich structure allows better angular properties (cell fixation).
Behaviour Under Dynamic Loading Conditions
This good response to impact is interesting, but pedestrians seldom impact at 90° to the surface, so dynamic tests have been carried out using a dome head impactor at 4.2 m/s. The results for the various PressLoad samples compare well with results for aluminium honeycomb, but can be improved - joining the flat tops of the cones to form a double sheet gives a double plateau owing to a two-stage crushing mechanism.
The first layer crushes to give the first plateau, and this crushed layer then acts as a skin during the second stage. Further investigations are being carried out regarding the multi-layer structure for the improvement of angle impact behaviour and the improvement of flexural and torsional stiffness.
PressLoad in a Sandwich Structure
Structural members made up of two stiff, strong skins separated by a lightweight core are known as sandwich panels. The separation of the skins by a structure such as PressLoad increases the moment of inertia of the panel with little increase in weight. This results in a structure that is light, but also resistant to bending and buckling loads.
Properties of Sandwich Structures
The mechanical properties (angular impact performances, flexural and torsional strength) can be optimised by the addition of skins (either on one or both sides), the design of a multi-layer structure or by the incorporation of soft material inside the structure. This addition of new structures (skins or additional layers) creates some new energy absorption modes, without changing the impact characteristics of the original PressLoad material.
The mechanical behaviour of sandwich panels depends on the properties of the face and core materials and on its geometry. For most applications a panel is required to have some minimum stiffness for a specified maximum loading, yet at the same time be as light as possible. The design of a sandwich panel can therefore be seen as an optimisation problem, where the structure must meet constraints on strength and stiffness while keeping its weight to a minimum. The design can be optimised by altering the core and skin thickness, core and skin materials, and the properties of the core itself.
The behaviour of Sandwich Structures under Test Conditions
A number of tests have been performed to determine the behaviour of materials when loaded. The edges of the sheet or panel were fully supported and the loading was applied at the central point. This represents how a head might impact a bonnet structure. The sheet materials have demonstrated a rapidly increasing force over the duration of the test, but the sandwich structures show comparatively less increase in deformation with increasing force. This would indicate lower peak forces, for example, in an impact situation.
Applications in vehicular Safety
Clearly, the material has many applications in vehicle safety. Specially shaped absorbers can be designed for interior protection, while panels can be used for floor and boot applications. There is also great scope for use in bonnet and bumper applications for pedestrian protection, the development of which is ongoing, requiring further research into:
- Development of new geometries
- Influence of the wall thickness
- Relationship between geometry and mechanical properties, to be studied with simulation tools
- Study of sandwich structure
- Oblique impact in single layers, sandwich, and with skins
- Influence of holes or other cuts in the structure.
Summary
In comparison to the classical energy absorbers, the PressLoad structure has many advantages, including excellent stress to weight ratio, its ability in angular energy absorption (especially for sandwich structures), its adaptable acoustic properties, exceptional flexural stiffness when sandwiched, excellent mechanical stability at very low-stress levels, particularly in compression. It is also easy to produce, either by cold forming, thermoforming or injection moulding and is available in many materials. For the car manufacturer it is a cost-effective solution as it is waterproof and can thus be used as a membrane. Furthermore, it is recyclable and safe. Perhaps most importantly, its predictable deformation characteristics reduce impact loading, making PressLoad a good hope for reducing pedestrian road fatalities.
|