Process Insights (GWI) manufactures and sells numerous fiber optic cables, probes and flow cells, designed for use in process environments, which can often involve explosive or combustible solids, gases and liquids. When deployed using incoherent sources, these devices are, for all practical purposes, intrinsically safe (IS).
However, there are two standards which can apply to the deployment of such devices. These are international standard IEC 60079-28 “Explosive Atmospheres – Part 28 Protection of Equipment and Systems Using Optical Radiation”1 and ANSI/ISA 12.27.01-2003 “Requirements for Process Sealing Between Electrical Systems and Flammable or Combustible Process Fluids”2.
In this article, it will be shown that under normal operating conditions, each of Process Insights’ SST3, O-SST4, and Multipurpose Flow Cells5 meet all the requirements of both of these standards.
Used to measure spectral features of process fluids, Process Insights’ fiber optic coupled probes and flow cells are typically used with either a spectrometer or photometer. Analysis of these spectral features then allows the chemistry or physical properties of the stream under test to be determined.
These measurements are typically carried out online, in real time, using an SST style insertion probe placed directly into a reactor or process pipe, with the process diverted through a loop to a flow cell.
The wetted region of the probe or cell is in close contact with the sample, as is the optical beam to be transmitted through the sample. The external portion of the probe is exposed to the process environment surrounding it, which may also be classified as hazardous. Under normal conditions, the optical beam is not in direct contact with the external environment, except in the case that there is a break in the fiber (i.e. a fault).
It must first be stated that the probes and fibers themselves contain no electrical hazards, and hence are intrinsically safe. It is only after being connected to an analyzer that light can enter the fibers and can be transmitted to the probe tip. As a result, if hazardous body certification (UL, ATEX, IECEx, CSA, etc.) is needed, it must be carried out in conjunction with the analyzer.
Process Insights’ probes have been used alongside Process Insights’ near-infrared (NIR) photometers (ClearView db) and spectrometers (Model 412, Lab 412, and NIR-O), in addition to instruments manufactured by others. In general, these third-party analyzers are near-infrared Fourier Transform (FT-NIR) spectrometers.
All of these analyzers use incoherent sources, for example tungsten-halogen bulbs, that operate by the principles of blackbody thermal radiation. Light from the source lamp is generally imaged through the end of the optical fiber, from which it is transmitted to the probe.
A collimated beam of light is then sent by the probe through the sample, from which it is injected back into a fiber. This returns the radiation to the analyzer, where it is ultimately detected.
For grating spectrometers, the light can either be dispersed before entering the fiber (pre-dispersed) or following its return from the probe (post-dispersed). In the pre-dispersed case (such as in GWI’s Model 412), monochromatic light is sent to the sample. This case will not be analyzed in this article as it is trivial compared to the post-dispersed case, in which white (also known as broadband) light is sent to the sample (such as in GWI’s NIR-O Spectrometer) which will be discussed below.
In the case of FT-NIR spectrometers, the light is encoded using an interferometer. This can also be pre- or post-encoded. In the majority of cases, FT spectrometers are pre-encoded as a means to reduce stray light interference. However, in both cases broadband radiation is sent to the probe. The pre-encoded case will now be discussed as it is the most common.
Photometers also fall in the post-dispersed configuration in most cases, with white light being sent out. Photometers will not be discussed in the following as they are not significantly different to the two cases above.
First, the IEC standard will be examined, followed by the ISA standard.
IEC 60079-28
The IEC 60079-28 standard was mainly developed for laser sources able to deliver significant energy into a fiber optic cable. In the following, the discussion will be limited to incoherent sources, namely tungsten-halogen lamps. These operate on the principle of black- or gray- body thermal radiation, which is defined by the well-known Planck Equation6.
The main potential hazard in this, is that the light source could potentially provide enough energy to ignite a combustible solid, liquid, gas, particle or droplet. Light from an incoherent source which is injected into a single strand fiber optic cable does not possess enough energy to ionize any target.
As a result, the only possible mechanism is through thermal heating by absorption of the radiation associated with the hazardous material or any other material in the beam.
Certain materials are broadband absorbers and can absorb all wavelengths received at their surface. Other materials possess selective absorption bands and broad regions which are spectrally transparent.
In these calculations, the former case is assumed. It should be noted that light from the end of a fiber optic cable rapidly diverges, and therefore the energy density significantly decreases with distance from the cable.
In the majority of cases, fiber optic cables used in process spectroscopy have a numerical aperture (N.A.) of 0.22. This means that the light diverges with a cone angle of 25° and hence any hazard decreases rapidly with distance7.
To be classified as intrinsically safe per the standard, the probe or flow cell optical energy density must be below 5 mW/mm2 at the point of interaction with the sample or hazardous medium.
For both probes and flow cells, the sample is made to flow between two windows, which are typically made from sapphire. Between the windows, there is a region in which a collimated beam of light transmits the sample, and where some or all of the light can be absorbed by the sample.
For GWI’s probes and flow cells, the collimated region is around 5 mm in diameter. At the point that the light exits the first sapphire window, the power density must be below the 5 mW/mm2 requirement.
In the following, the light source will be considered first. The source used in the majority of FT-NIR spectrometers as well as in GWI’s spectrometers, is an incandescent lamp with a filament made of tungsten. This filament is normally maintained at temperatures close to 2800 °K.
However, the worst-case scenario, in which the lamp is operating close to the absolute maximum of 3683 °K, the melting point of tungsten8 will be considered in the following calculations. The power density of a thermal source can be given by the Stefan-Boltzmann Law6:
Re = εσT4
Where Re is the radiant exitance in W/m2, ε is the emissivity, σ is the Stefan-Boltzmann Constant (5.67 x 10-8 W/m2/K4)8, and T is the source temperature in °K. In the case of tungsten, the emissivity has a maximum of 0.459.
Therefore, in the case of a 3683 °K source, Re = 4.69 W/mm2. The radiance (L) can then be calculated from the radiant exitance and defines the power per unit area per unit solid angle or steradian.
L = Re/π
Therefore the source radiance is L = 1.49 W/mm2/sr. This is the most common source for FT-NIR and grating spectrometers.
The calculations will now take two paths, describing the case for each instrument. Pre-encoded FT-NIRs lose half the light inside the interferometer.
In addition, the source optics will attenuate the beam as a result of reflection and transmission loses, however these values are unknown and depend on the optical geometry of the instrument.
The worst-case radiance for the FT can then be given by LFT = 0.76 W/mm2/sr. For GWI’s NIR-O spectrometer, the source optics design is known. The beam transits through a long pass filter with an efficiency of around 80%, and travels through eight (8) other optical surfaces, each with an efficiency of 96%.
This means that the source is attenuated by 0.8 * 0.968 = 0.58. From this the grating instrument radiance can be given by Lg = 0.86 W/mm2/sr.
The amount of light entering the fiber can be determined by the étendu of the fiber. The étendu is generally referred to as the A·Ω product, where A is the fiber’s cross-sectional area, and Ω is the acceptance solid angle of the fiber. For the area of the fiber, two cases can again be described.
Typically, FT-NIR spectrometers employ a 600 μm diameter fiber. GWI however uses 500 μm diameter fibers. Both of these fibers have an N.A. of 0.22 which can be used to define the acceptance solid angle. N.A. is the sine of the half cone angle, θ. The solid angle is then defined by the equation:
Ω = 2 π r (1 – cos(θ))
Considering a unit circle, r can simply be given by r = 1. Using a standard trig identity as well as the definition of the N.A., this equation becomes:
Ω = 2 π (1 – (1 – √(1 – N.A.2))
For both fibers, Ω = 0.154 sr. Therefore, for these two fiber choices, the étendu is A·Ω600 = 0.044 mm2·sr and A·Ω500 = .030 mm2·sr.
The total power, Pt, entering the fiber can be calculated as the product of radiance and étendu of the fiber as follows:
PtFT = LFT·A·Ω600 = 32.5 mW
Ptg = Lg·A·Ω500 = 26.1 mW
There remains one last correction that must be applied. Fused silica fiber strongly attenuates all radiation beyond 2300 nm, which can be > 1000 dB/km over 90% attenuation for a 10 m fiber run. It will now be assumed that the FT-NIR transmits all light between the visible and this wavelength.
Integrating the Planck Equation from UV to 2200 nm for a 3683 °K source provides a value of only 87.5% of the total energy, from which PtFT = 28.4 mW. The same long wavelength cut off will also apply to the grating spectrometer, but as noted previously, there is a long pass filter in the beam.
This filter removes all radiation below 950 nm. Thus, the integral can be considered to only be between 950 and 2200 nm, which is around 48.5% of the available light. From this, the power in the grating spectrometers fiber is Ptg = 12.7 mW.
If it is assumed that the fiber is lossless to the probe, the energy density in the optical beam through the sample can be calculated. For both SST probes and flow cells, the beam is about 5 mm in diameter, from which the power density (power per unit area) is
LpFT(3683 °K) = 28.4 mW/19.6 mm2 = 1.45 mW/mm2
Lpg(3683 °K) = 12.7 mW/19.6 mm2 = 0.66 mW/mm2
It was stated earlier that the intrinsically safe power density is 5 mW/mm2. Obviously, both of the cases considered here meet the specification as-is at the sample interface, even for this worst case scenario.
Had a more realistic blackbody temperature of 2800 °K been used, the values would have been
LpFT(2800 °K) = 0.43 mW/mm2
Lpg(2800 °K) = 0.28 mW/mm2
Which demonstrates that in both cases, at this realistic temperature, the values are an order of magnitude below the safe value.
One other consideration in the IEC standard is that the total power in the fiber must be below 35 mW, in case there is a break in the fiber. Again considering the worst case scenario, the total optical power in the fiber is PtFT = 28.4 mW and Ptg = 12.7 mW, both of which are well below the 35 mW requirement. For a 2800 °K lamp, these values drop to 9.4 mW and 5.4 mW respectively.
From this it can be concluded that GWI’s SST probes and flow cells satisfy the IEC 60079-28 standard for inherently safe (op-is) operation when used with a pre-encoded FT-NIR spectrometer or GWI’s post-dispersed NIR-O spectrometer, even in the case of two faults, lamp over voltage to burnout and a broken fiber or failed conduit.
ANSI/ISA 12.27.01-2003 Requirements for Process Sealing Between Electrical Systems and Flammable or Combustible Process Fluids
ISA 12.27.01-2003 essentially requires the presence of a secondary seal between the primary process seal and any conduit connections to the probe. The purpose of this seal is to prevent the migration of process fluid into the conduit system in the event that the primary seal fails.
For GWI probes and flow cells, the primary seal is located at the sapphire windows. This seal can be an O-ring, or a gold alloy braze, placed between the window and the stainless-steel probe body.
In the case of the SST probe and the O-SST probe, the fiber exits the probe via an SMA connector. These connectors are sealed to the probe body, either using O-rings or adhesives. For both probe designs, this seal mechanism has been tested to >3x the probe’s pressure rating. Therefore, the sealing method qualifies as a secondary seal under the ISA standard.
The construction of the flow cells is slightly different. In this case the primary seal is a double set of O-rings on the sapphire windows. The windows themselves are then held in place by the lens holders. Each lens holder has O-rings that are able to provide a seal between the lens holder and the cell body in addition to an O-ring around the lens itself.
It is this combination that makes up the secondary seal. This seal has also been proof tested to >3x the pressure rating of the cell.
Therefore GWI’s SST probes and O-SST probes, as well as GWI’s Multipurpose Flow Cells meet the ANSI/ISA dual seal requirement.
References
- “Explosive Atmospheres – Part 28 Protection of Equipment and Systems Using Optical Radiation”, International Electrotechnical Commission, 60079-28, 1st Ed. 2006-08.
- “Requirements for Process Sealing Between Electrical Systems and Flammable or Combustible Process Fluids”, American National Standards Institute/Instrumentation, Systems and Automation Society, 12.27.01-2003, approved 2 Feb. 2003.
- “Single-Sided Transmission (SST) Process Probe”, Process Insights, Product Literature, 1005-15-04.
- “O-Ring Single-Sided Transmission (O-SST) Probe”, Process Insights, Product Literature, 1009-12-11.
- “Multi-Purpose Process Flow Cell”, Process Insights, Product Literature, 1019-13-01.
- Halliday, D. and R. Resnick, Physics, John Wiley & Sons, Inc., 1968, Chapter 47.
- “Process Insights Fiber Optic Cable”, Process Insights, Product Literature, 1006-12-09.
- Handbook of Chemistry and Physics, R. C. Weast, Ed., CRC Press Boca Raton, FL, 67th Ed., p.B-40, F-188.
- American Institute of Physics Handbook, D. E. Gray, Ed., McGraw-Hill Book Co. New York, 3rd Ed., p.6-212.
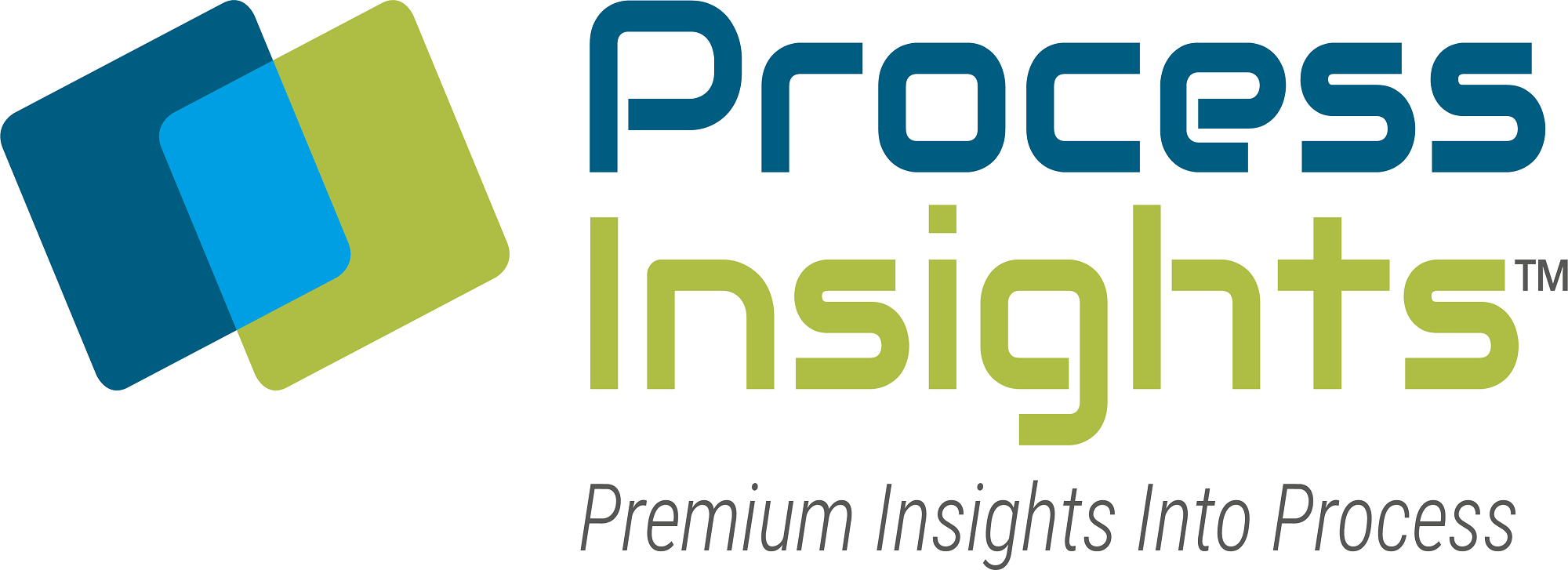
This information has been sourced, reviewed and adapted from materials provided by Process Insights – Optical Absorption Spectroscopy.
For more information on this source, please visit Process Insights – Optical Absorption Spectroscopy.