Phase change materials (PCM) are employed extensively in different fields of technology. Some applications include display devices, sensors, re-writeable data storage, and mechanical actuators.
Phase Change Materials
In many cases these materials are integrated into functional devices as thin films by means of different vacuum- or solution-based deposition methods.
To a large extent the details of the deposition conditions control the crystal structure and morphology of the PCM film, which in turn influence their phase change properties. Deposition processing parameters are not always adequate to optimize PCM properties, and post-deposition treatments are frequently needed.
Thermal annealing, which involves heating the whole sample, is the most frequent method to do this. Yet, this can cause undesirable alterations in other areas of the device. Solvent vapor annealing has been used more frequently in recent times, especially as a low-temperature alternative to thermal annealing in the field of organic electronics.
Solvent molecules which are in a vapor state can interact with the material’s surface and can also diffuse into it. This results in the decline of interactions between the building blocks (e.g. van der Waals bonds between molecules), which in turn get more mobile and the metastable material can thus develop into a thermodynamically more stable state: it can phase separate, crystallize, and so on.
However, solvent vapor annealing is complicated, and may also lead to undesirable outcomes, for example delamination, swelling and structural damage. Therefore, the annealing has to be optimized by modifying the temperature of the substrate, the concentration and nature of the solvent molecules, and also the length of the solvent exposure.
Linkam Scientific fabricates and investigates molecular PCMs in their laboratory, showing the so-called spin crossover (SCO) phenomenon. These transition metal complexes of iron, manganese, cobalt, or chromium ions can withstand reversible change between two phases (low spin and high spin) and so can function as active materials for storing and processing information and also for transducing different types of energy in actuators and sensors1.
Linkam Scientific recently deposited thin films of the SCO compound [Fe(HB(tz)3)2] (tz = triazolyl) successfully by thermal evaporation and found that a simple water-vapor annealing process can prompt drastic morphological and structural rearrangement within the as-deposited film2. The water vapor annealed films exhibited massively enhanced SCO characteristics, in terms of reproducibility, stability, and sharpness.
The Linkam instrumentation played a critical part in this research. the transformation between the as-deposited amorphous film and the stable crystalline film could be followed in-situ under controlled humidity and temperature conditions, in addition to ex-situ spectroscopic measurements to evaluate the efficiency of the process.
Method
The bulk powder of [Fe(HB(tz)3)2] was heated until 250 °C and evaporated at a rate of 0.003 nm/s at a base pressure of 2×10-7 mbar to grow films with a nominal thickness of ca. 100 nm on fused silica substrates.
Following this, by utilizing a Linkam THMS600-H stage in conjunction with an RH95 humidity controller, the as-deposited films were exposed to water vapor. By using a UV-VIS spectrophotometer, the UV absorbance of the films was observed in-situ throughout the solvent annealing process.
By utilizing the same Linkam stage together with Raman spectroscopy, the SCO properties of the annealed films were controlled. Using atomic force microscopy (AFM), the surface topography of the films was analyzed and their crystallinity was determined using x-ray diffraction (XRD).
Results
The crystallinity of the films of [Fe(HB(tz)3)2] considerably influences their UV absorbance (Fig. 1a), a phenomenon, which can be employed to observe the influence of solvent annealing in situ. Figure 1b shows experiments like these, which were carried out at different temperatures when increasing the relative humidity (Rh) from 43% to 90% in the Linkam THMS600-H chamber. Crystallization was noted only at low temperatures (< 40 °C) and the best results were gathered near room temperature.
Interestingly, at 25 °C when the relative humidity is increased gradually by the RH95 controller, a sharp jump of the absorbance (ca. 10 sec in duration) was seen. This corresponds most likely to a film crystallization process by growth and nucleation. This transformation was discovered to be irreversible, showing the formation of the thermodynamically stable film.
The films are hydrated after the water vapor annealing process, but in-situ Raman spectroscopic monitoring showed that gradual heating to 40 °C permits removal of the water content (Fig. 1c). A protocol was then determined (10 min annealing at 25 °C in Rh80% followed by 5 min annealing at 40 °C in ambient air) resulting in dehydrated, crystalline films of [Fe(HB(tz)3)2], which are smooth and homogeneous with no appreciable pinholes (Fig. 1d).
![(a) UV absorbance spectra of the pristine and water vapor annealed films. (b) In-situ monitoring the UV absorbance of [Fe(HB(tz)3)2] films when increasing the relative humidity from 43% to 90% at different substrate temperatures (25, 30, 40 and 80 °C). (c) Raman spectra of a film acquired subsequently (from bottom to top) on the pristine film, after water vapor annealing and after heating to 313 K. (d) AFM surface topography images of the initial (amorphous) and final (crystalline) films. Image size is 10×10 µm2. (Reproduced from Ref. 2 with permission from The Royal Society of Chemistry).](https://www.azom.com/images/Article_Images/ImageForArticle_17760_449747253375115768829.png)
Figure 1. (a) UV absorbance spectra of the pristine and water vapor annealed films. (b) In-situ monitoring the UV absorbance of [Fe(HB(tz)3)2] films when increasing the relative humidity from 43% to 90% at different substrate temperatures (25, 30, 40 and 80 °C). (c) Raman spectra of a film acquired subsequently (from bottom to top) on the pristine film, after water vapor annealing and after heating to 313 K. (d) AFM surface topography images of the initial (amorphous) and final (crystalline) films. Image size is 10×10 µm2. (Reproduced from Ref. 2 with permission from The Royal Society of Chemistry).
References and Further Reading
- G. Molnár, S. Rat, L. Salmon, W. Nicolazzi, A. Bousseksou, Spin Crossover Nanomaterials: From Fundamental Concepts to Devices, Adv. Mater. 2018, 30, 17003862.
- V. Shalabaeva, S. Rat, M. D. Manrique-Juarez, A.-C. Bas, L. Vendier, L. Salmon, G. Molnár, A. Bousseksou, Vacuum deposition of high-quality thin films displaying spin transition near room temperature, J. Mater. Chem. C 2017, 5, 4419.
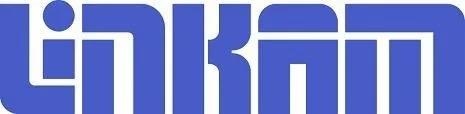
This information has been sourced, reviewed and adapted from materials provided by Linkam Scientific.
For more information on this source, please visit Linkam Scientific.