A routine challenge faced by manufacturers employing plastic parts is analyzing failed parts to determine the root cause of the failure. This article studies how several techniques can be used to analyze and confirm why a plastic part failed.
A manufacturer of precision optical equipment developed a plastic cover for a device with specifications for color and optical transmission, surface texture, and chemical composition.
In short, the cover was to be created from a polycarbonate – acrylonitrile butadiene styrene (PC-ABS) blend with enough titanium dioxide to give a marginally off-white color and an optical transmissivity of less than 0.01% T over a broad spectral range, from the UV into the near-infrared.
The opacity was needed to block ambient (room) light from reaching the optical device and hindering low light level measurements. Originally, each of the supplied parts fulfilled the specifications and the product delivered a satisfactory performance
A re-engineering project commenced to lessen costs and increase the competitiveness of the product. Quotations were then requested from different suppliers of various parts.
An alternative supplier underbid the earlier cover supplier, and the test parts satisfied all the requirements for opacity. As a result of this, the production was changed to include this new supplier.
After a short period of time, the product was failing serious performance tests. The failures were quickly traced to ambient light creating elevated backgrounds which strongly affected the low level optical measurements.
A visual inspection of the covers did not show obvious variations from the original, but different control experiments led to the failure being traced back to the new cover. A root cause analysis utilizing several techniques was carried out to efficiently discover and limit the issue.
Experimental Results
UV-Visible Spectroscopy
Diffuse transmission measurements of the earlier cover and failed cover were taken utilizing a Thermo Scientific™ Evolution™ 220 UV-Visible Spectrophotometer* and integrating sphere, as shown in Figure 1.
.jpg)
Figure 1. Evolution 220 UV-Visible Spectrophotometer* (left), and sample compartment integrating sphere accessory (right).
The cover by design included high quantities of particulates, which would quickly scatter any transmitted light. Due to this, transmittance was analyzed with an integrating sphere.
Parts of covers from both good and failing devices were positioned at the transmittance port of the sphere and spectra were gathered from 220 to 800 nm, resulting in the spectra demonstrated in Figure 2.
.jpg)
Figure 2. Diffuse Transmittance UV-Visible spectra of the failed cover (blue) and good cover (red), gathered with an Evolution 220 UV-Visible Spectrophotometer and integrating sphere accessory.
Virtually no transmittance was quantifiable through the good cover. In contrast to the failing cover, where a notable amount of transmittance through the visible part of the spectrum, greater than 7% T, was measured.
This evidently provided an explanation for the poor performance, - the light leak of the device under ambient conditions - but did not explain the root cause.
Thermogravimetric Analysis (TGA)
Small parts of both covers were then inspected with a TA Instruments™ thermogravimetric analyzer to discern bulk composition, with the results displayed in Figure 3.1
The samples were heated from ambient to 650 °C at 20 °C per minute under N₂ purge, then cooled to 550 °C, and heated again to 1000 °C at 20 °C per minute with air purge.
The first heating ramp under nitrogen pyrolyzes the organic component of the covers, and the last temperature ramp in air burns the rest of the organic components with only oxides of the inorganic content remaining.
The organic decomposition profiles of both covers were almost the same, showing that each one had the same plastic composition.
Although, the good cover contained leftover inorganic components representing 5.4% by weight, whereas the failed cover had an inorganic component of just 2.2% by weight. This showed an extensive variation in the amounts of inorganic filler between the covers and gave a strong hint as to the origin of the light leak.
.jpg)
Figure 3. Thermogravimetric analysis weight loss curves for the good cover (red) and failed cover (blue), demonstrating that the good cover has extensively higher inorganic content compared to the failed cover.
Infrared Analysis
Infrared spectra of small pieces of each cover were collected with the integrated diamond iS50 ATR on a Thermo Scientific™ Nicolet™ iS50 FTIR Spectrometer, displayed in Figure 4.
The built-in iS50 ATR on the Nicolet iS50 has an exclusive detector which allows the collection of combined mid- and far-IR ATR spectra down to 100 cm⁻¹. The capability of the iS50 ATR to gather spectra in the far-IR creates the simple measurement and discovery of inorganic fillers in plastic parts.
.jpg)
Figure 4. Nicolet iS50 FT-IR spectrometer with built-in diamond iS50 ATR, iS50 ABX Automated Beamsplitter exchanger, and sample compartment iS50 Raman accessory.
When integrated with the iS50 ABX Automated Beamsplitter exchanger on the Nicolet iS50 Spectrometer, mid and far-IR spectra can be immediately collected and stitched together utilizing a Thermo Scientific™ OMNIC™ Macros\Pro™ Visual Basic Program to give a single spectrum of a sample from 4000 to 100 cm⁻¹.2
The ATR spectra of the plastic parts, illustrated in Figure 5, were corrected with the advanced ATR correction algorithm3 in Thermo Scientific OMNIC Software.
The advanced ATR correction algorithm explains both relative intensity changes generated by sample penetration depth as a function of wavelength and also for peak shifts in the infrared spectra because of index of refraction variations between the ATR crystal and sample.
An examination of the infrared spectra of each plastic piece reveals the polymer composition to be similar, however the original plastic part has an elevated baseline below 800 cm⁻¹, and a sharp peak at 360 cm⁻¹, demonstrated in Figure 6, that are missing or very weak in the spectrum of the replacement part.
The peak at 360 cm⁻¹ is under the range of a common mid-IR spectrometer combined with a KBr beamsplitter. The iS50 ABX with a solid substrate far-IR beamsplitter allows the far-IR range to be accessible in this analysis, while high performance across the whole range is uncompromised.
There are further differences between the spectra which are highlighted through a spectral subtraction. The difference spectrum (Figure 5, bottom) indicates slight peak shifts in the polymer bands, showing a small polymer composition difference between each part, common when contrasting plastic parts made by alternate suppliers, but a critical spectral difference is also observed below 800 cm⁻¹.
A library search of the difference spectrum against a forensic library of automobile paint pigments and fillers,4 displayed in Figure 7, matches rutile, one of the crystalline forms of titanium dioxide, suggesting a formulation difference between both of the covers.
.jpg)
Figure 5. Advance ATR corrected infrared ATR spectra of the good plastic cover (top), failed plastic cover (middle), and difference spectra between the two (bottom).
.jpg)
Figure 6. Overlay of the advanced ATR corrected spectra of the good cover (blue) and failed cover (red), over the spectral region from 940 to 100 cm⁻¹. Note the elevated baseline and the absorbance band at 360 cm⁻¹ in the spectrum of the good cover that are missing or significantly reduced in the spectrum of the failed cover.
.jpg)
Figure 7. FT-Raman difference spectrum between the good and failed covers (blue), and top match from a library search against a forensic automobile paint pigment and fillers library (red), identifying a higher concentration of rutile (titanium dioxide) in the good cover.
FT-Raman Analysis
To verify the judgments taken from the infrared analysis, the two samples were also investigated utilizing the iS50 Raman sample compartment FT-Raman accessory on the Nicolet iS50 Spectrometer (displayed in Figure 4).
The iS50 Raman accessory fits into the sample compartment of the Nicolet iS50 FTIR Spectrometer and does not require an external module frequently seen in alternative FTIR spectrometer systems.
The iS50 Raman accessory allows for the simple gathering of Raman spectra with a near-infrared beamsplitter and an InGaAs detector mounted inside of the spectrometer.
FT-Raman spectra of the good and failed covers, in addition to the spectral difference spectrum between them, are shown in Figure 8. FT-Raman spectroscopy supports the collection of spectra into the far-IR region, accompanying the power of the Nicolet iS50 FTIR Spectrometer with the built-in iS50 ATR and ABX in gaining access to this region.
Again, the two spectra are highly alike, showing a comparable polymer composition, with slight differences in the spectra observable below 800 cm⁻¹, plainly seen in the difference spectrum.
A library search of the difference spectrum using a minerals Raman library5 is presented in Figure 9, establishing the difference between both plastic parts as rutile, confirming the identification from infrared analysis.
.jpg)
Figure 8. FT-Raman spectra of the good cover (top), failed cover (middle), and subtraction result between the two (bottom).
.jpg)
Figure 9. FT-Raman difference spectrum between the good and failed covers (top), and top library search result against a minerals Raman library (bottom), showing a higher concentration of rutile (titanium dioxide) in the good cover.
Summary and Conclusion
Ambient light reaching the device produced incorrect measurements for low light level measurements. Diffuse transmission measurement of the parts by UV-Visible spectroscopy established that the failed cover did not meet the specification for maximum transmittance.
Thermogravimetric analysis showed that the composition of the original cover had around 3% more, by weight, of an inorganic filler in contrast to the replacement cover.
Infrared ATR analysis over the mid and far-IR spectral regions demonstrated that the first cover had an extensively higher rutile (titanium dioxide) content than the second cover. The infrared results were verified by FT-Raman spectroscopy.
This study strongly shows that it is critical to have several tools available for root cause analysis. Most of the tools utilized can be found on the Nicolet iS50 FTIR Spectrometer. The Nicolet iS50 can collect multi-range spectra without compromise using the built-in iS50 ATR and iS50 Raman accessories.
The analyses given by the Thermo Scientific UV-Vis and FTIR instruments, in combination with thermogravimetric analysis, were conclusive in discovering the root cause failure of the plastic cover.
*This is now discontinued and replaced with Evolution One Plus UV-Vis Spectrophotometer. The ISA 220 accessory has not changed and is compatible with Evolution One Plus.
References and Further Reading
- Thermogravimetric results provided by Jeff Jansen, The Madison Group, 2615 Research Park Drive, Madison, WI, 53711.
- Mid-Far ATR iS50 collection program available upon request. Requires Nicolet iS50 FTIR Spectrometer configured with built-in diamond iS50 ATR, and ABX Automated Beamsplitter exchanger with KBr and solid substrate beamsplitter.
- Thermo Scientific Application Note 50581, Advanced ATR Correction Algorithm.
- An Infrared Spectral Library of Automobile Paint Pigments (4000–250 cm-1), developed by Dr. Edward H. Suzuki at the Washington State Police Crime Laboratory, downloadable from the SWGMAT.org website
- Downs R T (2006) The RRUFF Project: an integrated study of the chemistry, crystallography, Raman and infrared spectroscopy of minerals. Program and Abstracts of the 19th General Meeting of the International Mineralogical Association in Kobe, Japan. O03-13 Minerals 514 Raman Library
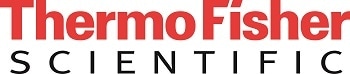
This information has been sourced, reviewed and adapted from materials provided by Thermo Fisher Scientific – Materials & Structural Analysis.
For more information on this source, please visit Thermo Fisher Scientific – Materials & Structural Analysis.