Infrared microscopy involves the examination of small samples, usually within the 10 to 100 μm range.
Spatial resolution becomes a significant concern on the scale. The infrared light's relatively long wavelengths set the theoretical limit for spatial resolution in infrared microscopy, but the actual achievable resolution depends on the infrared microscope's optical design and performance.
Obtaining high spatial resolution necessitates a careful balance of various elements in the infrared microscope's optical design, such as the collection optics (objectives and condensers), the number of apertures (their size and placement), and the overall mechanical stability of the microscope.
The design considerations notably impact one crucial aspect of spatial resolution, which is the diffraction of light. Several potential sources of diffraction exist within the optical path of an infrared microscope, and the design of Cassegrainian optics is a significant factor influencing the extent to which diffraction affects spatial resolution.1
The Thermo Scientific™ Nicolet™ RaptIR™ and RaptIR+ FTIR Microscopes employ high numerical aperture (NA) Cassegrainian optics featuring an innovative design that effectively minimizes optical aberrations and reduces the impact of diffraction.
The unique design enables the RaptIR+ to achieve excellent spatial resolution without using multiple apertures, thus avoiding the reduction in throughput (intensity) associated with additional apertures. The data provided here demonstrates that the RaptIR+ can achieve a spatial resolution of 5 μm.
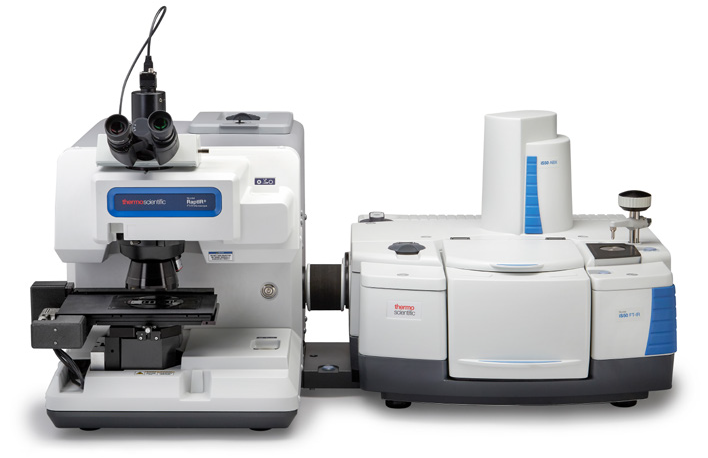
Nicolet RaptIR+ FTIR Microscope coupled with the Nicolet iS50 FTIR Spectrometer. Image Credit: Thermo Fisher Scientific – Materials & Structural Analysis
Experimental
Data collection was carried out using a Nicolet RaptIR+ FTIR Microscope, which was equipped with a 4X optical objective for a wide field of view, facilitating the easy location of areas of interest.
Additionally, the RaptIR+ featured innovative 15X Cassegrainian optics, comprising both the objective and condenser, to provide higher-magnification visible images and collect the necessary infrared data. The instrument was connected to a Thermo Scientific™ Nicolet™ iS50™ FTIR Spectrometer.
All measurements were obtained in the form of horizontal line maps, taken with 1 μm intervals and utilizing a 5 μm x 5 μm aperture. Two different types of spatial resolution measurements were conducted during the study. The first sample employed was an Edmund Optics USAF 1951 optical target.
The sample consisted of a dark USAF 1951 pattern deposited on a chrome-coated glass slide measuring 2” x 2”. Data collection was conducted in reflection mode, with the chrome-coated glass serving as the background. For collecting spatial resolution data, elements 1-5 from USAF 1951 optical target group 6 were utilized.
The second sample type consisted of a flat piece of topaz, featuring a clean, straight edge. The sample was thin enough to be used in transmission experiments and exhibited a well-defined, sharp O-H peak at 3649 cm-1.
Data collection for the sample took the form of a knife-edge experiment, where the transition from no sample peak to full intensity served as the spatial resolution measurement criterion.
Results
The reflection measurements on the USAF 1951 target involved conducting line maps along the vertical lines of the first five elements in group 6. Notably, the thickness of the lines in Element 5, measuring 4.92 μm, closely approached the 5 μm target for the investigation.
Transitioning from the chrome-coated portions of the target to the dark lines resulted in a shift in baseline intensity towards lower reflection values. Figure 1 displays plots of reflection values at 4000 cm-1 as the mapping crosses the lines in the patterns. From the data collection, two distinct metrics were computed.
The first metric was the full width at half maximum (FWHM) of the peaks associated with the dark lines, providing an indicator of line width. The second measurement was contrast, representing the difference in reflection between the peaks and valleys related to the dark lines.
As the lines became thinner and more closely spaced, the contrast decreased, yet maintaining contrast was necessary for distinguishing the lines from each other, with the Rayleigh criteria for image contrast being 26.4%.
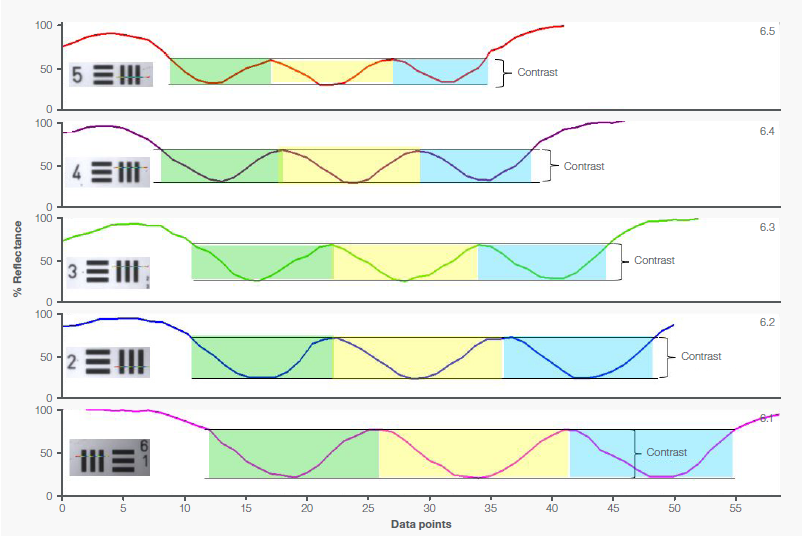
Figure 1. Plots of intensities (reflection) at 4000 cm-1 versus map steps across the USAF 1951 optical target - group 6 patterns (elements 1-5). Image Credit: Thermo Fisher Scientific – Materials & Structural Analysis
Table 1 summarizes the data for all five group 6 elements. As measured from the FWHM values, the line widths closely matched the expected widths and fell well within the experiment's margin of error.
Contrast values exceeded the Rayleigh criteria for spatial resolution, signifying sufficient contrast for line resolution, which confirms the RaptIR+'s capability to achieve a 5 μm spatial resolution.
The second spatial resolution test was a transmission knife-edge test, where data collection involved traversing the sample's edge with 1 μm steps. The transition's sharpness served as a measure of spatial resolution.
A thin topaz piece was employed due to its formation in thin, straight-edged sheets. The topaz sample exhibited a distinct O-H peak at 3649 cm-1, as depicted in Figure 3.
Figure 4 illustrates a plot of the peak area of the O-H peak while moving along the sample's edge. The figure includes the second derivative of the curve, which defines the transition's boundaries.
The spatial resolution was calculated based on the second derivative limits, occurring at 13% and 88% of the full peak values, resulting in a spatial resolution of 5 μm. However, it is important to note that this does not guarantee complete spectral isolation.
Suppose there are infrared-active materials on both sides (e.g., a layered polymer cross-section). In that case, small residual contributions from the previous layer may still be present on the 5-μm scale in the spectra of the new material.
Achieving more stringent spectral isolation would affect the transition limits and spatial resolution values.
Conclusion
The findings presented in the report demonstrate that the RaptIR+ is capable of achieving a spatial resolution of 5 μm. The level of resolution was observed in both the reflection and transmission experiments.
Notably, the Rayleigh criteria for contrast in the reflection experiment and the second derivative limits in the topaz knife-edge experiment both support the feasibility of achieving a 5 μm spatial resolution.
The results make it evident that RaptIR microscopes offer commendable spatial resolution without the need for multiple apertures, thus avoiding the inherent reduction in throughput associated with an extra aperture.
Table 1. Contrast and Full Width at Half Height (FWHH) results from the plots of the reflection intensity at 4000 cm-1. Source: Thermo Fisher Scientific – Materials & Structural Analysis
Group 6
Element |
Actual line
thickness (μm) |
FWHH
(μm) |
Difference
(μm) |
Contrast
(%) |
6-1 |
7.81 |
8.1 |
-0.29 |
54 |
6-2 |
6.96 |
7.0 |
-0.04 |
47 |
6-3 |
6.20 |
6.4 |
-0.2 |
42 |
6-4 |
5.52 |
5.7 |
-0.18 |
36 |
6-5 |
4.92 |
5.0 |
-0.08 |
28 |
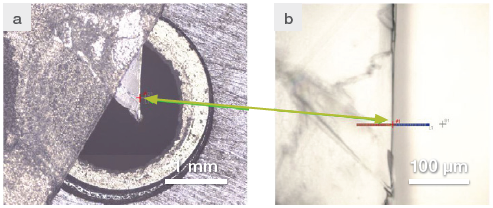
Figure 2. Visual images from the topaz sample. (a) Visual image collected with the 4X objective on the RaptIR, (b) Visual image collected with the 15X objective on the RaptIR. Image Credit: Thermo Fisher Scientific – Materials & Structural Analysis
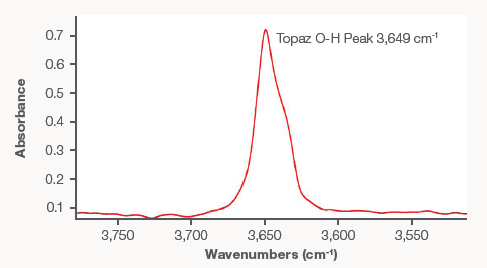
Figure 3. Oxygen-Hydrogen peak (3649 cm-1) from the topaz sample. Image Credit: Thermo Fisher Scientific – Materials & Structural Analysis
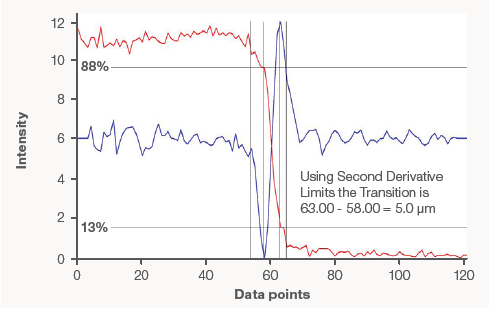
Figure 4. Transmission knife-edge measurements using the topaz sample. The plot of peak area (3649 cm-1 peak) as a function of map steps across the edge of the sample is in red and the second derivative of the curve is in blue. Image Credit: Thermo Fisher Scientific – Materials & Structural Analysis
References
- Koichi Nishikida, Spatial Resolution in Infrared Microscopy and Imaging, Application Note 50717 Thermo Electron Scientific Instrument Corporation (2004).
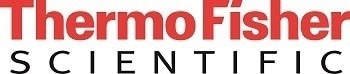
This information has been sourced, reviewed and adapted from materials provided by Thermo Fisher Scientific – Materials & Structural Analysis.
For more information on this source, please visit Thermo Fisher Scientific – Materials & Structural Analysis.