There have been important technological advancements made in the nondestructive testing (NDT) industry with total focusing method (TFM) capable inspection devices becoming more common. In terms of phased array ultrasonic testing (PAUT) technology, the TFM approach represents a significant step forward. However, TFM and its relationship to full matrix capture (FMC) is still confusing for some PAUT practitioners, along with the differences between conventional PAUT and TFM/FMC processing.
This article provides a basic introduction to TFM imaging for those who are familiar with PAUT imaging. Aspects related to ultrasound mode conversion are set aside here to keep things concise and clear.
Fundamental Differences Between Conventional PAUT and TFM
A multi-element probe is used in both PAUT and TFM, which emits pulsed ultrasound waves in the test piece and records the time-trace of echoes (waveform). An image of reflectors in the inspected piece is then produced by assembling these waveforms. An ultrasound image can be viewed as a stack of sub-images called frames. An arrangement of A-scans (amplitude versus time) captured at different angles makes up a sectorial scan in PAUT.
In the definition of the sectorial scan, individual A-scans act as frames. These frames are processed in the fastest way possible with the PAUT strategy to display and refresh the global image in real-time.
The strategy of signal acquisition and frame processing is the fundamental difference between conventional PAUT and the TFM.
Conventional PAUT Imaging
An S-scan is used here to demonstrate the frame acquisition process in PAUT. The S-scan is made up of individual frames that correspond to A-scans captured at various angles in the piece. A group of elements, known as the aperture, fire and record at the same time during acquisition.
To steer the ultrasound beam at the required angle, and to focus it at the desired depth in the piece, each element has a delay applied. The refracted angle and focus depth are then used to define each frame. This means that the number of discrete angles composing the global image is the total number of frames to acquire.
A limited amount of acquisitions are required with PAUT, which is advantageous. “Physical summation” in the material of individual transmitters’ acoustic amplitude results in the transmitted beams.
The rapid summation capacity of front-end electronics is used to synthetically obtain reception beams, meaning that images obtained through PAUT can be displayed very quickly. One disadvantage of PAUT is that the frames are only focused at a constant depth. In comparison to identical reflectors appearing in the focal zone, reflectors located outside of the focal region appear blurry and somewhat larger.
This resolution problem is solved with the total focusing method (TFM). The amplitude is displayed over focal lines at multiple depths with the TFM, producing a highly resolved image everywhere, not just over a single depth line.
FMC–An Acquisition Strategy
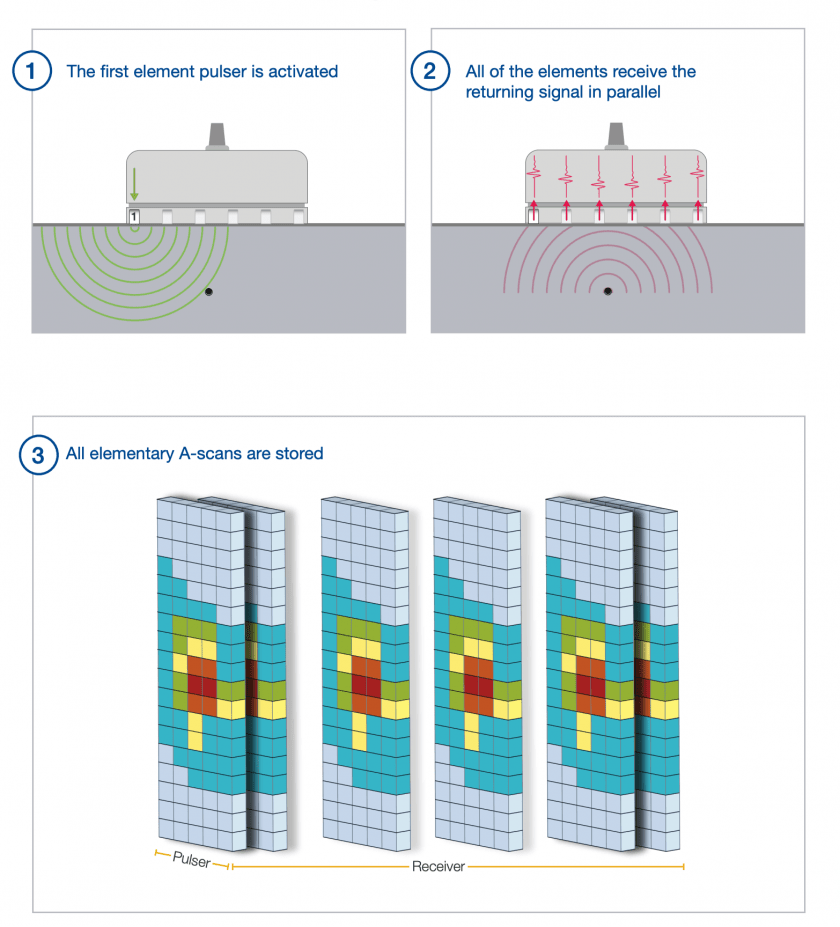
Image Credit: Evident Corporation
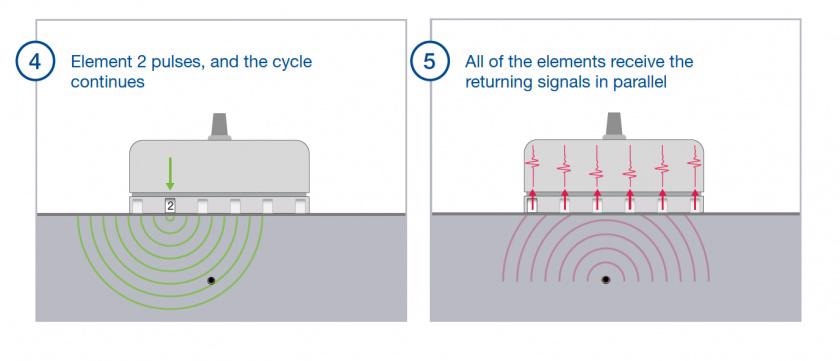
Image Credit: Evident Corporation
TFM–Image Reconstruction
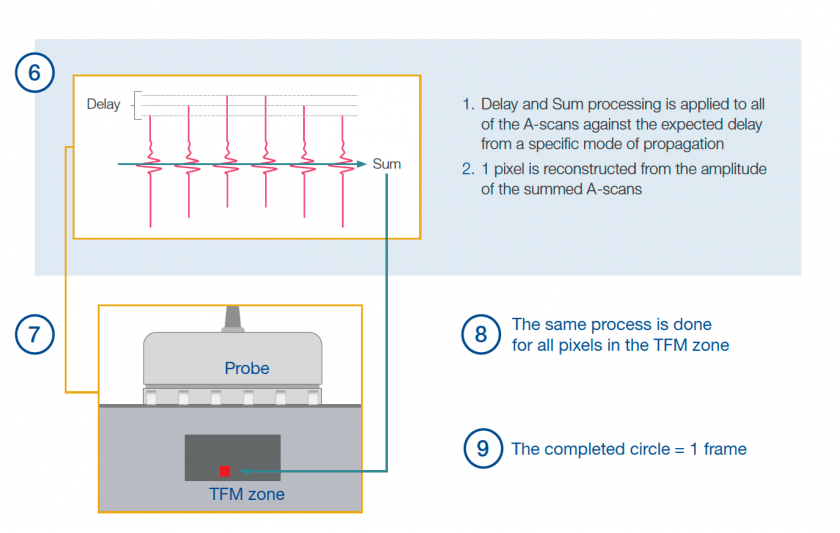
Image Credit: Evident Corporation
The time taken to generate a TFM image would be dramatically increased if the PAUT acquisition strategy were to be used for the total focusing method. With the TFM, the number of discrete angles required to generate an S-scan is a lot lower than the number of pixels constituting an image.
As an example, 100 acquisitions would be required for an S-scan that is swept through 100 angles, whereas 10,000 acquisitions would be required for a TFM image of 100 × 100 pixels.
As illustrated in Figure 2, another acquisition strategy is used to avoid this problem, whereby frames are calculated post-processing. A set of focal laws corresponding to each pixel position is required for this strategy, as well as a set of raw fundamental waveforms called full matrix capture (FMC).
Ultrasound beams can be synthetically generated both in transmission and reception and focused at each pixel location, as these two elements allow fundamental waveforms to be delayed and summed appropriately. As such, the generated image is “focused everywhere.”
All the waveforms between all individual pairs of probe elements are acquired with FMC. The best focusing result for a given probe design is generally achieved with the full aperture of the probe. Here, to build the FMC, the number of acquisitions needs to be equal to the number of elements of the probe.
All information regarding sound propagation between each element of the probe is contained within the FMC, including reflections at interfaces and scattering by flaws. FMC can be used to reconstruct any type of PAUT acquisition, including a sectorial scan, plane wave imaging (PWI), dynamic depth focusing (DDF), etc.
The number of acquisitions required to generate an image using the FMC acquisition process is approximately equal to PAUT. However, significant storage capacity, transfer bandwidth, and processing power are all required to store the individual FMC data sets. Obtaining the TFM/FMC results can be slower than PAUT depending on the electronics of the device used.
Illustration of Differences Between PAUT and TFM Images on an Experimental Case
The differences between PAUT and TFM imaging are illustrated through a setup in which a linear phased array (PA) probe is used to scan identical side-drilled holes (SDHs) that are vertically distributed in a steel block.
Presented below are a PAUT S-scan (a) and a TFM image (b) obtained using OmniScan™ X3 flaw detector with the same inspection configuration.
A unique focal distance of 20 mm (represented by a red dashed line) is used to acquire each frame in the S-scan. SDHs located in the focal area are shown with similar amplitude and size.
In comparison to a shorter focal distance, the area where the image resolution is optimal is larger, explaining the above result. SDHs appear distorted and with a significantly lower amplitude the farther away they are from the focal depth. For a more uniform sizing of all the SDHs, several images with various focal distances are required.
Ultrasound beams are focused at each pixel in the TFM image (b). As shown here, every one of the SDHs appears with an optimal resolution, and only a single image is required to adequately size SDHs located over a larger depth range.
Lateral distortion is still observed, however, for SDHs located at the extremities of the electronic focusing capabilities as this distortion is intrinsic to PA imaging.
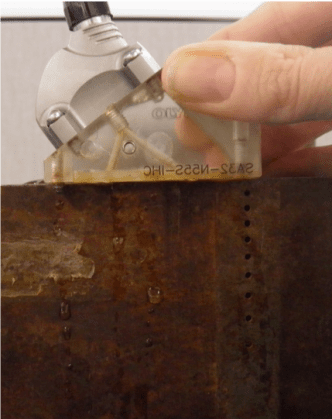
Image Credit: Evident Corporation
Summary of the Advantages Provided by TFM/FMC Acquisition
The nature and number of frames composing the images is the main difference between the TFM and PAUT.
Frames are one-dimensional signals or A-scans in PAUT
and only real-time, front-end electronics summation acts as post-processing. Furthermore, frames are acquired and presented on the fly in PAUT.
In contrast, the TFM frames are zero-dimension data points from a focused beam at each pixel’s coordinates. Compared to PAUT, there is a larger number of frames to process. To produce synthetic focalized beams in post-processing, the FMC acquisition process is required.
In comparison to an image produced with PAUT, the entire image is displayed with an optimal resolution in the TFM, which is only highly resolved in the focal area of the beam. However, it is worth noting that, when using the total focusing method, the electronic focusing capacity is a limitation carried over from phased array imaging.
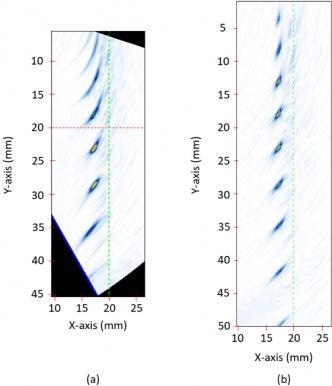
Image Credit: Evident Corporation
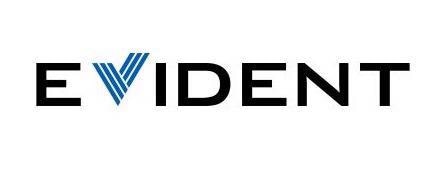
This information has been sourced, reviewed and adapted from materials provided by Evident Corporation.
For more information on this source, please visit Evident Corporation.