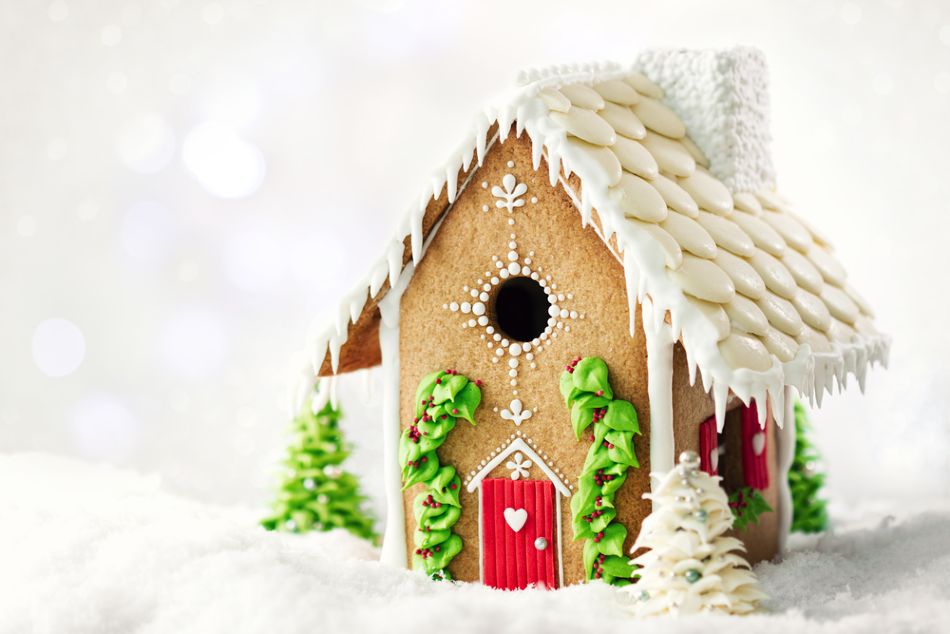
Image Credit: Ruth Black/Shutterstock.com
In 2019, in a tour de force of skill and advanced technology, a researcher at the Canadian Centre for Electron Microscopy at McMaster University in Hamilton crafted the world’s smallest gingerbread house. The word ‘crafted’ is accurate, as the level of detail is exquisite.
The gingerbread house has richly textured walls, a stout brick chimney, a wreath over the door and a doormat in the shape of the Canadian flag. But the most astonishing feature of the house is its size - it is less than 10 micrometers (a micrometer or µm is 10-6 m) in height (comparable to the size of a dust particle or pollen) and is carved out of a single crystal of silicon.
The scientists have placed the minute house on top of a tiny snowman carved out of nickel-cobalt lithium aluminate alloy used as a cathode material in the modern lithium-ion batteries. A human hair placed next to the composition emphasizes the microscale of the objects.
Sculpting the microscopic structure out of high-tech materials is an elegant demonstration of the remarkable powers of the modern scanning electron microscopes (SEM).
Canadian researcher builds gingerbread house just 1/10th of a hair in length
Video Credit: CBS 17/YouTube.com
Electron Beams
In the SEM, the sample surface is ‘illuminated’ with a focused beam of electrons in the same way that light or a focused beam of photons is used in the optical microscopes. The electron beam is formed by using electromagnetic fields and is scanned in a raster pattern across the sample surface.
Find out more about microscopy equipment
The electrons that are scattered back (back-scattered electrons or BSE), or knocked out of the topmost atomic layer of the specimen (secondary electrons or SE), are detected and analyzed to provide a pixel-by-pixel image of the specimen surface with a resolution on a sub-nanometer level (a nanometer or nm is 10-9 m).
Click here to find out more about electron beam sources
Ion Beams
Electrons are not the only charged particles that can be accelerated and focused by using electromagnetic fields. In a focused ion beam (FIB) instrument, which is very similar to an SEM, a beam of ions (atoms with a net electrical charge due to the lack or excess of electrons) is used to probe the sample surface.
In modern FIB microscopes ions of Gallium (Ga) are used - these are 130,000 times heavier than the electrons and carry much higher momentum. Because of that, the focused ion beam can directly modify or ‘mill’ the specimen surface, by knocking out entire atoms from the sample surface, with nanometer accuracy. By carefully controlling the parameters of the ion beam, it is possible to perform very accurate nanomachining to produce minute components or to remove unwanted material.
Dual-Beam FIB-SEM Instruments for Nanofabrication
A FIB becomes even more powerful when it is combined with an SEM, as this provides an immediate, high-resolution SEM imaging of the FIB-modified surface. It is a FIB-SEM tool that the Canadian researchers used to carve out the microscopic gingerbread house.
The every-day applications of these instruments are diverse: from micro- and nano-sectioning of hard materials (e.g. metals, glass, ceramics) or multi-layered semiconductor structures (that cannot be easily sliced by cutting tools) to the preparation of ultrathin electron-transparent lamellae that can be investigated by transmission electron microscopy (TEM), and to the fabrication of micro- and nano-scale structures. The ion beam spot size is approximately 5–10 nm, thus enabling nanometre-size features to be created.
Nanoscale Tomography
Another ‘specialty’ application of the FIB-SEM technique is the nano-tomography of objects with complex internal structure (such as semiconductor devices, biological cells, and metal alloys), where the elucidation of the spatial distribution of the structural features, the number of features per unit volume, and the feature connectivity is of great interest.
The process involves sequential slicing of the specimen (by using the ion beam) with subsequent imaging of the corresponding cross-section by the electron beam. Combining the individual cross-sections creates a three-dimensional representation, or a tomogram, of the internal structure of the specimen.
In 2017, a group of researchers from Howard Hughes Medical Institute in the United States managed to bring this technique to its limits in terms of spatial resolution, automated continuous data acquisition (over several months), and advanced data analysis algorithms.
This allowed mapping of the interconnectivity of the neurons in mammalian neural tissue and in Drosophila (small fruit fly) brains at an unprecedented level of detail.
Elemental Analysis
Nanotomography can be combined with energy dispersive X-ray (EDX) analysis that can provide information about the building materials in the specimen. When interacting with high-energy electrons (the imaging beam in an SEM), the atoms of the sample emit X-rays with specific energy (depending on the occupancy of the electron shells in the atom) that are the material’s fingerprint.
By detecting the energies of the characteristic X-rays that come out of a sample of unknown composition, it is possible to identify all the different chemical elements in the sample.
The SEM-FIB-EDX technique allowed the performance of direct chemical analysis of exposed cells (by FIB ‘milling’) in cancerous tissue of mouse urinary bladder epithelium, as demonstrated in 2019 by a research group from the University of Ljubljana in Slovenia.
Electron Beam Patterning and Lithography
The electron beam of an SEM can also be used to create nanoscale patterns and structures. Unlike the direct modification of the sample surface by the FIB, the electron beam lithography (EBL) involves a thin polymer layer (resist) coated onto a solid substrate. The resist layer is sensitive to the electrons and changes its properties in the areas exposed to the electron beam.
The patterns written by the beam into the resist can be developed (positive or negative) and subsequently transferred onto the substrate by etching or deposition methods. In 2019, researchers from the University of Nottingham in the UK used EBL to create the world’s smallest periodic table, just 14 µm by 7 µm in size, on a polished silicon substrate. Each element symbol is about 100 nm across, while the width of the lines outlying the symbols is less than 30 nm.
This nanoscale periodic table is a clear demonstration of the capabilities of the tools and techniques that scientists use in their every-day work in the pursuit of a deeper understanding of the structure-property-function relationships in a wide range of materials and processes. Engaging projects like this help to develop scientific curiosity in the wider audience.
Sources and Further Reading
Disclaimer: The views expressed here are those of the author expressed in their private capacity and do not necessarily represent the views of AZoM.com Limited T/A AZoNetwork the owner and operator of this website. This disclaimer forms part of the Terms and conditions of use of this website.