Granular materials and fine powders are commonly utilized in industrial applications. For the control and optimization of processing procedures, such materials need to be characterized in a precise way. The methods of characterization are linked to the characteristics of the grains, for example, granulometry, morphology, chemical composition, etc., and to the bulk powder behavior, for example, flowability, density, blend stability, electrostatic properties, etc.
However, with regards to the physical behavior of a bulk powder, the majority of the methods utilized in R&D or quality control laboratories are created on previous measurement procedures. Throughout the last decade, GranuTools has modified these methods in order to meet the current requirements of R&D laboratories and production departments.
Specifically, the measurement procedures have been made automated, and rigorous initialization techniques have been developed to gain results that are reproducible and interpretable. Additionally, image analysis techniques have been used to enhance the precision of the measurements.
Several industries are now utilizing GranuTools instruments range in a variety of areas. For example, additive manufacturing, food processing, pharmaceuticals, and bulk material handling. This article primarily focuses on additive manufacturing.
Metallic and polymer powders are largely utilized in Additive Manufacturing (AM) processes with the involvement of powder bed like powder bed fusion (SLM, SLS, …) or binder jetting. Throughout these kinds of operations, successive thin layers of powder are generated using a ruler or using a rotating cylinder.
Then, every layer is partially sintered or melted with an energy beam for the production of the parts. The layer thickness establishes the vertical resolution of the printer; a thin layer results in a superior resolution. For a thin layer to be obtained, the powder is as fine as possible.
Unfortunately, when there is a decrease in the grain size, there is an increase in the cohesiveness and a decrease in the spreadability. The flowability needs to be efficient enough to get homogenous successive powder layers. The quality of the parts built with AM is therefore directly linked to the powder flowing properties.
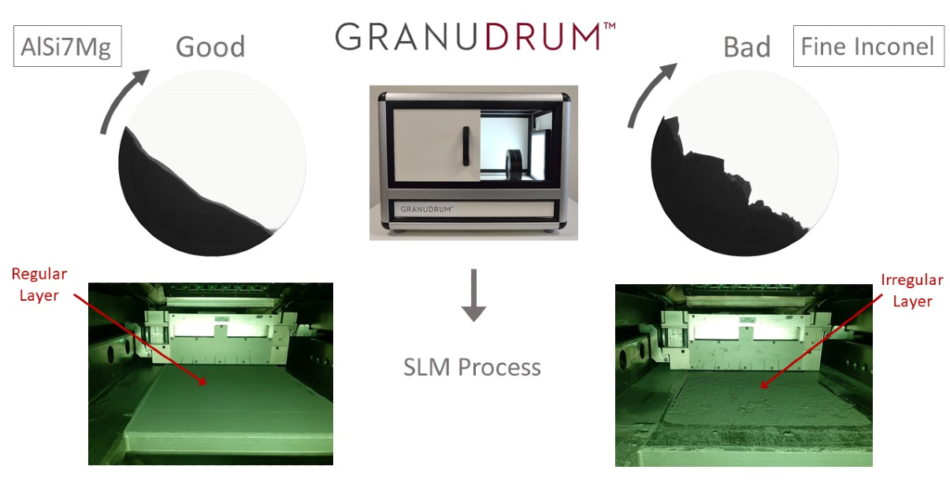
Figure 1. The general principle of how to relate GranuDrum measurements to powder performance inside an SLM printer.
Usually, visual observation of layer homogeneity is the only solution for operators to quantify the spreadability of powders throughout the recoating. However, linking the powder characteristics to its spreadability during the recoating operation before should offer an increasingly cost-effective method of classifying and choosing the beset powder and recoating speed combinations.
The main objective of this article is to show how characterizing macroscopic properties of metallic powders can be linked to their spreadability within SLM printers. The spreadability of four metal powders has been established using the GranuDrum. This is an automated rotating drum measurement technique, with a cohesive index measurement that has been proven to quantify the spreadability of the powder throughout the recoating process (Yablokova et al. 2015).
A new technique combining in situ measurements inside an SLM printer and image processing have been developed to quantify the homogeneity of the powder bed layers during recoating. The overall principle of this study is outlined in Figure 2: a low cohesive powder which displays a smooth powder/air interface in the rotating drum will create a more homogeneous layer within the printer.
In contrast, a cohesive powder with bad flowing properties will display an irregular interface in GranuDrum and a non-homogeneous layer on the bed throughout the recoating.
Three recoater speeds have been investigated to quantify the recoating velocity influence on the powder spreadability.
To enhance the printing duration it is interesting to reduce the recoating time by increasing the recoater velocity. However, processing powders at a higher shear rate can result in a rise of cohesiveness, i.e. shear-thickening behavior. Consequently, a compromise needs to be determined between recoater speed and spreadability.
GranuDrum
The GranuDrum instrument is a powder flowability measurement method that is automatized. It is based on the rotating drum principle. A drum; a horizontal cylinder with transparent sidewalls, is half-filled with the sample of powder. The drum then rotates around its axis at an angular velocity with a range from 2 rpm to 60 rpm.
Snapshots are taken with a CCD camera, approximately 30 to 100 images separated by 1s, for each angular velocity. The air/powder interface is identified in each snapshot with an edge detection algorithm. After, the average interface position and the fluctuations surrounding this average position are calculated.
Then, for every rotating speed, the flowing angle, also known in previous studies as ‘dynamic angle of repose’, αf is calculated from the average interface position and the dynamic cohesive index σf is calculated from the interface fluctuations. Generally, a low value of the flowing angle αf corresponds to a good flowability.
The flowing angle is impacted by a large range of parameters. For example, the friction between the grains, the cohesive forces (van der Waals, electrostatic and capillary forces) between the grains and the shape of the grains.
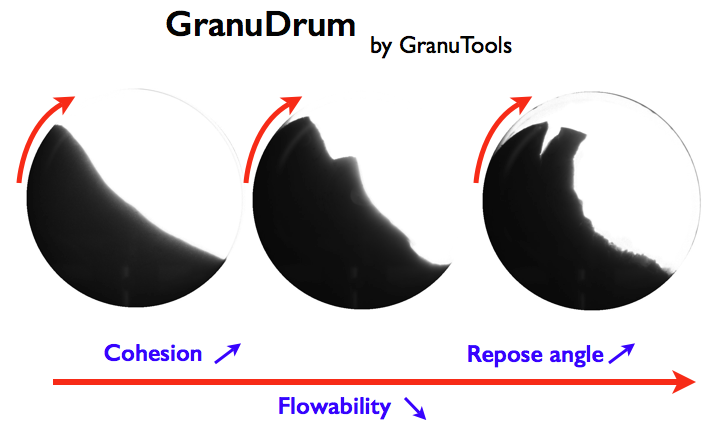
Sketch of GranuDrum measurement principle
The dynamic cohesive index σf is just linked to the cohesive forces between the grains. A cohesive powder results in an intermitted flow, and a non-cohesive powder results in a regular flow. Consequently, a dynamic cohesive index nearest to zero corresponds to a non-cohesive powder.
When the powder cohesiveness rises, the cohesive index rises in accordance. As well as the measurement of both the cohesive index σf and the flowing angle αf as a function of the rotating speed, GranuDrum permits measurement of the first avalanche angle and the powder aeration throughout the flow. The cohesive index is focused on in this article.
Powders Description
Four metallic powders supplied by the Sirris were chosen for this research: two Nickel alloys (Inconel®, Inconel® fine) and two Aluminium alloys (AlSi7Mg06, Scalmalloy®).
Table 1 summarizes particle size distribution:
Powder |
d10 (µm) |
d90 (µm) |
Inconel® Fine |
3,6 |
22 |
Inconel® |
14 |
46 |
AISi7Mg06 |
20 |
63 |
Scalmalloy® |
20 |
59 |
Visual Characterization
Experimental evaluations of the recoating performance of the powders inside the SLM printer have been completed with in situ observation of layer homogeneity achieved by Sirris in Belgium. After the recoater performs each deposition of a layer, a picture is taken by a camera situated within the printer. An initial visual characterization can be formed on the basis of these pictures.
- AlSi7Mg06: homogeneous layer, with only small waves orthogonal to the recoater displacement. Additionally, the layer is more homogeneous at the highest speed (160 mm/s) demonstrating an increase of spreadability with recoating speed.
- Inconel®: homogeneous layer, with only extremely light parallel waves emerging at 160 mm/s. This powder signifies a good spreadability.
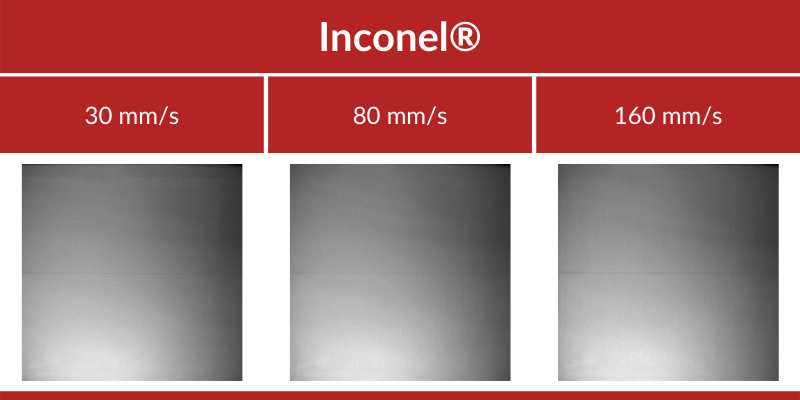
- Scalmalloy®: achieve a lower spreadability when compared with AlSi7Mg06 and Inconel®. Certainly, at 80 mm/s strong orthogonal waves are seen together with light parallel waves. These parallel waves are more noticeable at 160 mm/s, therefore showing that Scalmalloy® perform better at low speed.
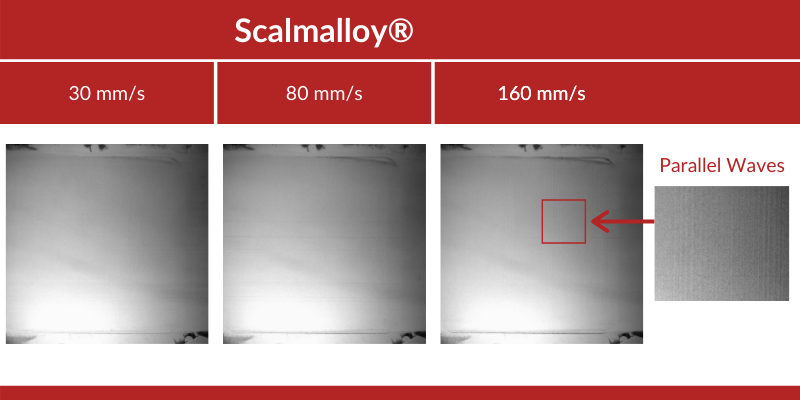
- Inconel® fine: Highly inhomogeneous layers. The deposition of a homogeneous layer is impossible even at low speed (30 mm/s). This powder undoubtedly displays the worst spreadability.
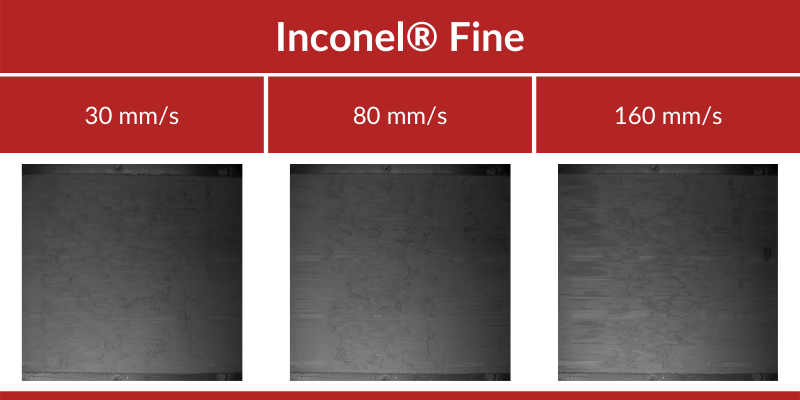
When looking at these visual observations, it is clear that these powders demonstrate very different recoating performance.
GranuDrum Analysis
Experimental Protocol
For GranuDrum experiments, powders were added to the measuring cell immediately following box opening. The powder quantity was around 50 ml. Each powder was analyzed in ambient conditions of 35% RH and 23 °C.
GranuDrum velocities were explored from 2 to 60 rpm. 40 pictures were taken for each velocity to enhance the accuracy and repeatability of the measurement. Powders were measured three times to observe the reproducibility of the result. Error bars are displaying the standard deviation with respect to the average value.
Experimental Results
Figure 2 shows the Cohesive Index as a function of the GranuDrum increasing rotating speed, which can be linked to the recoater speed (as seen in Appendix 1). The Cohesive Index is related to the fluctuations of the interface (powder/air) position triggered by cohesive forces (Van der Waals, Electrostatic, and Capillary).
Therefore, it permits to quantify powder spreadability. For a simple comparison, Figure 2 also indicates the three recoater speeds used in the in situ SLM printer measurements.
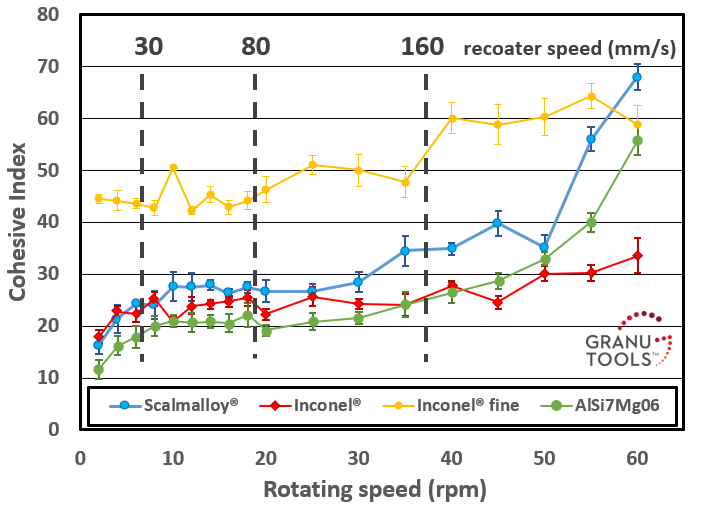
Figure 2. Cohesive Index versus Rotating drum speed. A shear-thickening behavior is observed for all the powders, especially above 40 rpm.
Discussion
Over the investigated velocity range, the Inconel® fine displays the higher cohesive index and therefore the lower spreadability. This is a result of the smallest particle size of this powder, resulting in a higher cohesiveness.
The AlSi7Mg06 powder displays superior spreadability at low speeds and is closely followed by the Inconel®. Scalmalloy® has a higher cohesive index in comparison to AlSi7Mg06 and Inconel®, and thus a lower spreadability.
Additionally, all the powders have a shear-thickening behavior for rotating speed above 40 rpm. This performance is characterized by an increase of the cohesive index with speed. Consequently, the spreadability is predicted to decrease when the recoater speed is increased.
In particular, powders AlSi7Mg06 and Scalmalloy® are affected in comparison to the Inconel® which displays a moderate shear-thickening. This behavior presents a restriction for the upper limit of recoating speed, and if higher, the spreadability will not be good enough to generate homogeneous layers of powder.
These results suggest that to attain the fastest recoating speed while also maintaining a good spreadability within the printer, a recoating speed near the highest recoating velocity (160 mm/s) selected in this study appears to be the optimal compromise for these powders.
Spreadability Assessments
Experimental Protocol
The powder spreadability classification received by the GranuDrum measurements can be compared with the real performance inside the printer. Yablokova et al. have previously demonstrated that the spreadability inside the printer is directly linked to the cohesive index, but only with a visual investigation.
Next, a more qualitative approximation of the homogeneity of layers is shown. The powder spreadability is experimentally assessed with a camera situated inside an SLM printer. Following each recoater operation, a picture is taken. For the identical recoater speed, 15 layers are created, and consequently, 15 pictures are taken as well.
The GranuLayer software has been specifically created by GranuTools to quantify the inhomogeneity of the powder bed surface within the printer. The “interface fluctuation” is introduced as a measure of the inhomogeneity of the successive layers gained by the recoating. The measurement principle is as follows:
- Every picture is analyzed independently of others. The size of the picture is 1200 x 1200 px.
- Horizontal and vertical pixel intensity profiles are taken out at discrete positions of the picture, as shown in figure X.
- An average “smooth” profile is computed for each position, as shown in figure X.
- Then, interface fluctuation is calculated on the basis of the deviation around the averaged profile and then averaged overall positions.
- The procedure is repeated for all the images, and the interface fluctuation is average over the entire set of pictures.
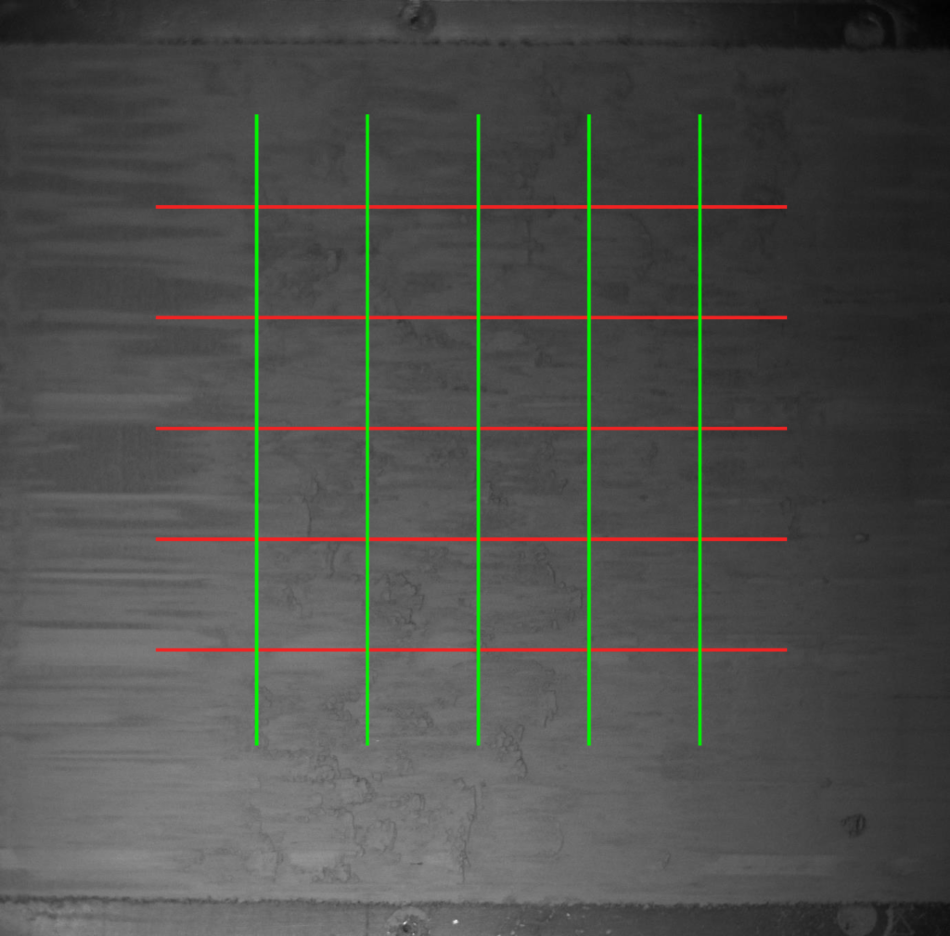
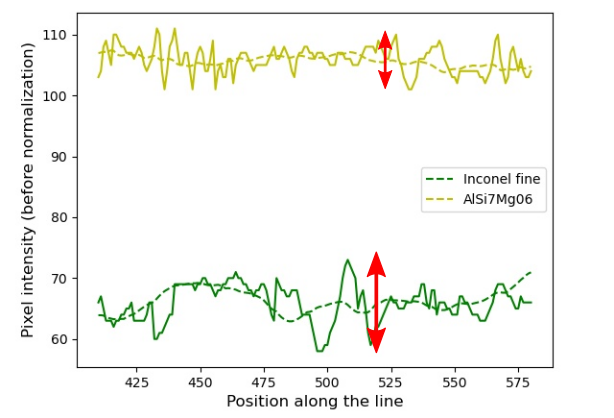
Figure 3. Top: horizontal and vertical lines from which pixel intensity profiles are extracted. Bottom: pixel intensity profile (plain) and average profile (dashed) used to compute the interface fluctuation.
Experimental Results
Figure 4 shows the interface fluctuation in comparison to the recoater speed obtained with the image processing analysis:
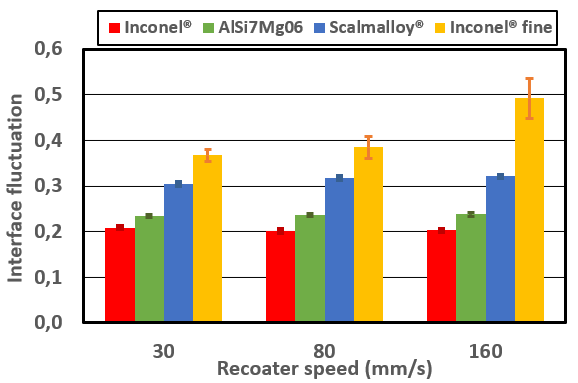
Figure 4. Interface fluctuations as a function of recoater speed (in mm/s). The recoater speed effect is more pronounce for the Inconel® fine, as observed also in GranuDrum.
Discussion
Now, the homogeneity of layers is quantified with more precision with the interface fluctuation parameter. Inconel® and AlSi7Mg06 displays the lower interface fluctuation, and therefore the best spreadability inside the printer for all recoater speeds.
Due to these powders being only marginally influenced by the recoater speed, processing at 160 mm/s seems to be the optimal recoating velocity. These results are well correlated with the cohesive index attained with the GranuDrum.
Certainly, the shear-thickening behavior is weak under 160 mm/s. Scalmalloy® displays a higher interface fluctuation because of the appearance of parallel waves throughout recoating, in accordance with a higher measured cohesive index. Finally, the Inconel® fine that has the higher cohesive index exhibits a higher interface fluctuation signifying a poor recoating performance for all speeds.
These results demonstrate that homogeneity of layers attained throughout the recoating is directly linked to the cohesive properties of the powder. Certainly, a more cohesive powder (higher cohesive index) results in a higher interface fluctuation because of a lower spreadability.
Conclusions
- Investigations into the spreadability of four metal powders have been achieved and comparisons have been made against their real performance inside SLM printers.
- Cohesive index measurements have been correlated with an interface fluctuation parameter calculated from pictures taken within the SLM, relating the powder cohesiveness to the homogeneity of layers attained throughout the recoating process.
- The classification of powders seen with GranuDrum can be correlated with the homogeneity of layers. The capability of a powder to generate regular layers is thus directly linked to cohesiveness.
- Consequently, powder characterization with GranuDrum and GranuPack offers a helpful classification of powders, in addition to determining the best recoater velocity to increase the printer process quality.
Acknowledgments
GranuTools would like to thank the Sirris (Olivier Rigo) for providing the powders and for performing all the 3D printing experiments.
Bibliography
- Cascade of granular flows for characterizing segregation, G. Lumay, F. Boschin, R. Cloots, N. Vandewalle, Powder Technology 234, 32-36 (2013).
- Combined effect of moisture and electrostatic charges on powder flow, A. Rescaglio, J. Schockmel, N. Vandewalle and G. Lumay, EPJ Web of Conferences 140, 13009 (2017).
- Compaction dynamics of a magnetized powder, G. Lumay, S. Dorbolo and N. Vandewalle, Physical Review E 80, 041302 (2009).
- Compaction of anisotropic granular materials: Experiments and simulations, G. Lumay and N. Vandewalle, Physical Review E 70, 051314 (2004).
- Compaction Dynamics of Wet Granular Assemblies, J. E. Fiscina, G. Lumay, F. Ludewig and N. Vandewalle, Physical Review Letters 105, 048001 (2010).
- Effect of an electric field on an intermittent granular flow, E. Mersch, G. Lumay, F. Boschini, and N. Vandewalle, Physical Review E 81, 041309 (2010).
- Effect of relative air humidity on the flowability of lactose powders, G. Lumay, K. Traina, F. Boschini, V. Delaval, A. Rescaglio, R. Cloots and N. Vandewalle, Journal of Drug Delivery Science and Technology 35, 207-212 (2016).
- Experimental Study of Granular Compaction Dynamics at Different Scales: Grain Mobility, Hexagonal Domains, and Packing Fraction, G. Lumay and N. Vandewalle, Physical Review Letters 95, 028002 (2005).
- Flow abilities of powders and granular materials evidenced from dynamical tap density measurement, K. Traina, R. Cloots, S. Bontempi, G. Lumay, N. Vandewalle and F. Boschini, Powder Technology, 235, 842-852 (2013).
- Flow of magnetized grains in a rotating drum, G. Lumay and N. Vandewalle, Physical Review E 82, 040301(R) (2010).
- How tribo-electric charges modify powder flowability, A. Rescaglio, J. Schockmel, F. Francqui, N. Vandewalle, and G. Lumay, Annual Transactions of The Nordic Rheology Society 25, 17-21 (2016).
- Influence of cohesives forces on the macroscopic properties of granular assemblies, G. Lumay, J. Fiscina, F. Ludewig and N. Vandewalle, AIP Conference Proceedings 1542, 995 (2013).
- Linking compaction dynamics to the flow properties of powders, G. Lumay, N. Vandewalle, C. Bodson, L. Delattre and O. Gerasimov, Applied Physics Letters 89, 093505 (2006).
- Linking flowability and granulometry of lactose powders, F. Boschini, V. Delaval, K. Traina, N. Vandewalle, and G. Lumay, International Journal of Pharmaceutics 494, 312–320 (2015).
- Measuring the flowing properties of powders and grains, G. Lumay, F. Boschini, K. Traina, S. Bontempi, J.-C. Remy, R. Cloots, and N. Vandewalle, Powder Technology 224, 19-27 (2012).
- Motion of carbon nanotubes in a rotating drum: The dynamic angle of repose and a bed behavior diagram, S. L. Pirard, G. Lumay, N. Vandewalle, J-P. Pirard, Chemical Engineering Journal 146, 143-147 (2009).
- Mullite coatings on ceramic substrates: Stabilisation of Al2O3–SiO2 suspensions for spray drying of composite granules suitable for reactive plasma spraying, A. Schrijnemakers, S. André, G. Lumay, N. Vandewalle, F. Boschini, R. Cloots and B. Vertruyen, Journal of the European Ceramic Society 29, 2169–2175 (2009).
- Rheological behavior of β-Ti and NiTi powders produced by atomization for SLM production of open porous orthopedic implants, G. Yablokova, M. Speirs, J. Van Humbeeck, J.-P. Kruth, J. Schrooten, R. Cloots, F. Boschini, G. Lumay, J. Luyten, Powder Technology 283, 199–209 (2015).
- The flow rate of granular materials through an orifice, C. Mankoc, A. Janda, R. Arévalo, J. M. Pastor, I. Zuriguel, A. Garcimartín and D. Maza, Granular Matter 9, p407–414 (2007).
- The influence of grain shape, friction and cohesion on granular compaction dynamics, N. Vandewalle, G. Lumay, O. Gerasimov and F. Ludewig, The European Physical Journal E (2007).
Appendix 1: Relation Between Drum Rotating Speed and Process Speed (in mm/s)
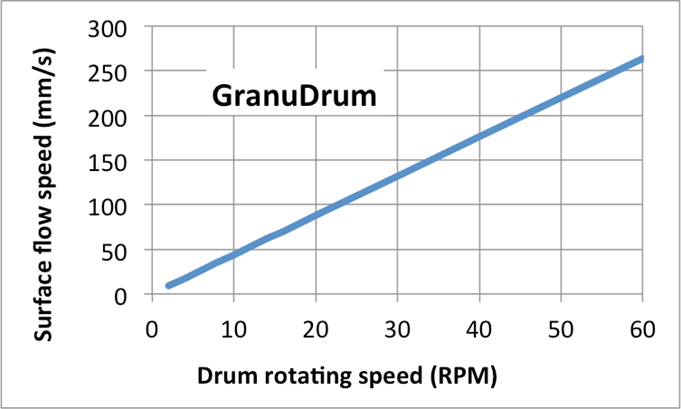
Figure 5. Relation between drum rotating speed and process speed (in mm/s).
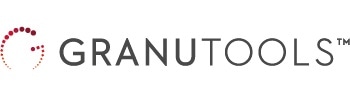
This information has been sourced, reviewed and adapted from materials provided by Granutools.
For more information on this source, please visit Granutools.