The number of ions belonging to different elements within a sample can tell a lot. In beverages and foods, different ions have specific limits established to ensure a minimum nutrient content is reached and to safeguard health.
Increased levels of certain heavy metal ions in environmental water sources or soil can suggest that a pollution event has happened. Ultrapure water is used in several manufacturing processes and if the presence of any ions is above a certain threshold it indicates that either the filter system requires maintenance, or the source water is not suitable for use.
There are many different ways to carry out ion measurement, for example, atom absorption spectroscopy (AAS), ion chromatography (IC), or inductively coupled plasma optical emission spectrometry (ICP-OES).
These are all widely used, well-established methods within analytical laboratories. However, initially, this is relatively costly. Using an ion-selective electrode (ISE) for ion measurement is a promising alternative to these expensive techniques.
This article discusses the challenges that could be encountered when applying standard addition or direct measurement, and the ways analysts can overcome them to achieve better confidence with this type of analysis.
Introduction
In 1889, Walther Nernst developed the Nernst equation, which allowed the evolution of measuring pH using potentiometric sensors to become possible. Determining pH was the only analysis for which this equation was of importance for almost 70 years.
In the early 1960s, it was remarked that cuts of lanthanum fluoride crystals displayed very high selectivity towards fluoride ions1, 2. This led to the building of the first ion-selective electrode next to the pH electrode.
From then, discovering new glass, crystal, polymer materials, and gas-permeable membranes gradually led to the development of ion-selective electrodes. A few of these different ISEs are discussed in Table 1.
Table 1. Overview of assorted ion-selective electrodes and their capabilities3.
Membrane Material |
Ions |
Crystal |
Ag+, Cu2+, Pb2+, Br-, Cl-, CN-, F-, I-, S2- |
Polymer |
Ca2+, K+, Na+, Surfactants, NO3- |
Glass |
Na+, H+ |
Gas permeable membrane |
NH4+ |
Sometimes ion-selective electrodes can make performing measurements challenging, but analysis of a specific ion of interest can be attained easily if certain essential points are taken into account.
Some general tips for potentiometric ion measurement are given in the following sections, as well as specific ones for direct measurement and standard addition.
General Hints
Performing ion measurement is very easy. The manufacturer’s instructions must be followed in order to ensure both the accuracy of the analysis and long-term user satisfaction. An overview of some common questions and problems is given in the following points.
Measuring Range of Electrode
There is a specific measuring range for each type of electrode, which normally falls within a linear range of around six decades of mol/L. Figure 1 shows the linear range for the case c in which there are no interferences present. For this region, concentration is proportional to the signal and follows the Nernst equation.
The curve flattens and the potential difference becomes smaller in the high and low-level concentration regions, which is displayed in Figure 1 for case c. The linear range is reduced by interfering ions reduce causing the measuring range to decrease.
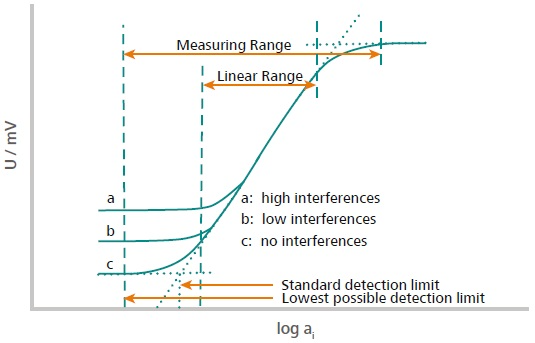
Figure 1. This illustration explains how the measuring range and the linear range are defined. The measuring range is defined from the two points located in the high and low concentration range where no potential change is observed anymore. This means either the concentration is too low, or the sensor is saturated. The linear range is defined as the range where the signal evolves linearly with the activity (concentration) of the ion according to Nernst. Image Credit: Metrohm AG
Therefore, in order to obtain reproducible and precise results measuring in the linear region is recommended.
If the sensor is calibrated in this confined range, however, there is also the possibility of an ion concentration measurement in the flattened, non-linear region by direct measurement.
Electrodes usually have a measuring range of 10-6 to 1 mol/L. The measuring range could increase up to 8 mol/L and down to 10-8 mol/L depending on what ion-selective electrode is used.
An analyst needs to be sure that the electrode can measure in the concentration range of the sample before ion measurement is selected as the method of choice.
Interfering Ions
The first ion-selective electrode built was the pH electrode. An excellent linear range of 14 decades is exhibited by the electrode, and it is also selective for H+. Alternatively, the majority of other ion-selective electrodes are not very selective for the ion being measured.
Reversible and irreversible are the two different types of interfering ions. Irreversible interfering ions either bind to the membrane material and react, or they form complexes and precipitate with the measuring ion, making it unavailable for further analysis.
If the irreversible interfering ion is reactive towards the membrane, its presence needs to be avoided or the ISE will be destroyed. Reversible interfering ions show cross-sensitivities due to having similar electronic properties and size as the measuring ion. These can also bind to the membrane material and contribute to the signal, yielding erroneous results.
Due to cross-sensitivities being so frequent in ion measurement, the Nernst equation was expanded to the Nikolsky equation:
U = U0 ± ((2.303 × R × T) / (n × F)) × log(ai + Σkij × ajnm)
U: measured potential between ISE and reference electrode in mV
U0: measured potential between ISE and reference electrode at c(ion) = 1 mol/L in mV
R: universal gas constant (R = 8.314 J/(mol∙K))
T: temperature in Kelvin
F: Faraday constant (96485 C/mol)
n: electrical charge of the ion
ai: activity of the ion to be measured
kij: selectivity constant (ion to be measured / interfering ion)
aj: activity of interfering ion
m: electronical charge of the interfering ion
This equation is currently implemented in the majority of ion meters for direct measurement.
Conditioning of the Electrode
Normally, ion-selective electrodes are supplied dry and independent of the membrane material used. It is essential to condition the electrode before activating the measuring membrane.
Conditioning ensures that the equilibrium of the measuring ion in the membrane is maintained, giving the possibility of accurate measurement. The proposition is to use an ion standard solution with a conditioning solution of c(ion) = 0.01 mol/L concentration.
A stable equilibrium of the measuring ion in the membrane is required in order to obtain reliable ion measurements, and so, in between measurements, the ISE should also be conditioned.
Here, the concentration of the measuring ion within the sample influences the conditioning solution. The manufacturer documentation for specific ion-selective electrodes or applications can provide more information regarding the correct conditioning solution.
Total Ionic Strength Adjustment
Ion measurement depends not on the concentration of the measuring ion in solution, but on the activity of this, and thus the total ionic strength adjustment is required. The concentration of ions in the solution, as well as the pH, which has an effect on the dissociation of specific molecules, causes this parameter to vary.
Because of this, it is necessary to at least maintain a constant ionic background, which can be attained by adding an ionic strength adjuster (ISA).
A fixed pH value is also needed depending on the measured ion. For this, a total ionic strength adjustment buffer (TISAB) would be used. Normally, the relatively high concentration of salt contained by the ISA and TISAB solutions does not interfere with the measurement.
Specific ISA and TISAB solution recommendations can be found in the manual provided for the ion-selective electrode or online.
Table 2. Examples of ISA/TISAB solutions for different ionic measurements and information regarding where to find the analysis procedure.
Cleaning of the Electrode
The electrode is cleaned thoroughly after each measurement using deionized water. Organic solvents may reduce the lifetime of ion-selective electrodes with crystal membranes and can destroy ion-selective electrodes with polymer membranes, and so they should not be used for cleaning.
When very low concentration samples are being measured, the electrode should be allowed to stand for 10 minutes in deionized water or ISA / TISAB before beginning the next measurement.
If not, the ions bound to the electrode membrane material from the previous measurement could diffuse out and as a result, alter the signal and the obtained result of subsequent samples.
Diffusion could lead to an enhancement in the concentration of the measured ion and in doing so, contamination of the deionized water. Hence, the deionized water should be replaced prior to each measurement.
Storage of the Electrode
The electrode’s storage requirements are dependent on the type of the electrode and the time period of storage (Table 3). The manual that comes with the ion-selective electrode outlines the proper storage instructions.
Table 3. Recommendations for the storage of different ISE types.
Membrane material |
Short storage period |
Long storage period |
Polymer |
Dry |
Dry |
Polymer, combined |
In c(ion) = 0.01–0.1 mol/L |
Dry, with some residual moisture |
Crystal |
In c(ion) = 0.1 mol/L |
Dry, with protective cap |
Glass |
In c(ion) = 0.1 mol/L |
In deionized water |
Electrode Lifetime
An ISE’s lifetime depends on the sample matrix, electrode maintenance, and membrane type. Acceptable electrode maintenance involves proper conditioning, frequent cleaning, and storage as has been previously defined in this article. It is also necessary to regularly replace the electrolyte of the reference electrode.
As previously mentioned, the membrane of the ion-selective electrode can react with the sample matrix. The electrode manual normally lists any substances that need to be avoided. For example, the polymer membrane of ISEs can be destroyed by organic solvents, and the crystal of the lead ISE suffers interference in the presence of acetate.
Polishing the membrane regularly can regenerate it in ion-selective electrodes with a crystal membrane. The ISE manual usually contains the suitable polishing material and procedure3. If they are well-maintained and if lasting sample matrix influences are avoided, these types of electrodes can last many years of laboratory use.
Polymer membrane electrode lifetime is more limited when compared to those with crystal membranes.
With time, a loss in performance results from the aging of the polymer membrane. This type of ISE normally has a best before date, up until which they have their best performance. The electrode can be used up until this date, and Metrohm can promise this performance for an additional six months after this date.
Generally, if subject to normal laboratory use, the mean lifetime for polymer membrane electrodes is approximately six months.
The electrode should always be handled with care and the membrane should not be touched with bare fingers, as a sweat or fatty film deposit from the skin can negatively impact the electrode’s performance.
Measuring Modes
There are two different ways to perform potentiometric ion measurement: standard addition and direct measurement. These measuring principles both have advantages and disadvantages. Table 4 lists these for comparison.
The correct analysis method can be chosen properly when taking into account knowledge of the sample’s properties and the frequency of your analysis.
Standard Addition
In ion measurement by standard addition, the sample must first be pipetted into a beaker, before ISA/TISAB and then several increments of the standard are added.
The potential is measured following every addition. After this, ion concentration is calculated iteratively from the volume increment and potential difference using the method of least squares.
There are two common types of standard additions. In one, defined amounts of standard can be added to the sample, or the device itself can automatically add the corresponding amount of standard until a formerly defined potential difference is attained.
Defined potential difference is the second form of standard addition and this is recommended, as it obtains more reliable results.
Table 4. Advantages and disadvantages of standard addition and direct measurement.
Standard addition |
Direct measurement |
Advantages |
- Matrix independent
- No calibration necessary
- Only a single standard is needed
- Nearly no user interaction necessary
- Electrode is tested with each measurement
|
- Fast measurement (parameters determined quickly, on average in approximately 60 seconds of measuring time)
- Good reproducibility, also at lower concentration ranges
|
Disadvantages |
- Poor reproducibility in low concentration ranges
- Longer determination times (parameters
- determined within approximately 300 seconds of measuring time)
|
- A series of standards for calibration must be prepared
- Matrix-dependent
- Change of electrode properties is only seen during calibration
|
The standard addition method of ion measurement is chosen as soon as the matrix is unknown and could consist of interfering ions that influence the measured signal.
The total volume of standard added should be between 10 and 90% of the buret volume, and there should be at least four additions (Figure 2). The buret must not be refilled.
To avoid dilution falsifying the result, the added volume should not surpass 25% volume. Another factor that must be avoided is temperature differences between the sample solution and the standard solution.
Another critical point to consider is the potential difference.
As much standard as required should be added to obtain a voltage difference of 12 mV per addition at minimum. The device itself calculates the result iteratively.
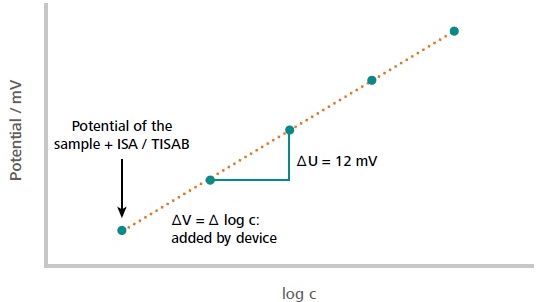
Figure 2. Example of a typical standard addition curve. The first measuring point corresponds to the measured potential of the sample solution (including auxiliary solutions). The subsequent measuring points correspond to the measured potential after each addition of the standard. The initial concentration of the sample is calculated from the measured potential difference in dependence of the added volume increment. The dilution of the sample by auxiliary solutions is considered in the calculations as well. Image Credit: Metrohm AG
The following points must be checked if the results are not reproducible:
- Per addition, how much standard is dosed?
- While adding standard, is the solution stirred?
- How tight is the dosing unit?
- Does the tubing contain air bubbles?
- Between the additions, does the potential measurement reach a stable potential?
- Is the attained slope acceptable?
Direct Measurement
If the sample matrix only consists of a few interfering substances and is similar for all samples, this type of ion measurement is carried out. Furthermore, for samples containing low ion concentrations analysis using direct measurement is more suitable.
When measuring via standard addition, these samples cause problems: the curve flattens and proper evaluation is no longer possible (non-linear range).
A calibration must be carried out beforehand to determine the concentration of the ion in the sample.
The calibration standard concentrations should be chosen so that they cover the expected concentration of the ion in the sample.
The calibration standards comprise of a particular concentration of the measuring ion and the same proportion of deionized water to ISA/TISAB to be used in the determination itself afterward.
In order to reduce the influence of carryover, it needs to be made sure that the standards from the lowest to the highest concentration are measured.
To carry out the analysis, the standard is pipetted into a beaker, the corresponding amount of ISA/TISAB is added, and the sensor is immersed.
Then, the result is shown directly in the selected concentration unit. It is also important not to forget to take into account the dilution created by adding ISA/TISAB.
The subsequent points can be checked if the results are non-reproducible:
- For the measuring solution and for all of the standards, is the amount of ISA/TISAB the same?
- For the sample and standard measurements, is the same ratio of ISA/TISAB to sample/standard plus water used?
- Is the slope of the calibration attained satisfactory?
- Were the standards measured in the defined / correct order?
- Is the sample concentration range sufficiently covered by the calibration range?
- Between measurements was the electrode properly conditioned?
General Points to Consider for Ion Measurement
- What is the age of the electrode? Polymer electrodes, in particular, often age rapidly. Depending on the type of electrode, Metrohm proposes there to be a maximum shelf life of 2 years.
- Whether the sensor was properly conditioned, as otherwise the signal and the corresponding results tend to drift.
- The measured value drift is important and a drift maximum of 1 mV/min should be used.
- Whether the standard is freshly prepared. Some standards tend to change their concentration with time.
- By immersing the sensor in c(ion) = 1 ∙ 10-4 mol/L and c(ion) = 1 ∙ 10-3 mol/L, the electrode can be checked. The electrode is acceptable and can be used if the difference in voltage corresponds to no less than 47.3/z* mV (at 25 °C) (80% of the Nernst Slope), z* being the charge of the ion.
Summary
Potentiometric ion measurement by direct measurement or by standard addition is a good alternative to other more expensive techniques like IC, AAS or ICP-OES.
The determination is fast and reliable when respecting the intended use, measuring range, and conditions of storage.
References and Further Reading
- Oehme, F. Ionenselektive Elektroden, Grundlagen und Methoden der Direktpotentiometrie; Dr. Alfred Hüthig Verlag GmbH: Heidelberg, 1986.
- Cammann, K. and Galster, H. Das Arbeiten mit ionenselektiven Elektroden - Eine Einführung für Praktiker; Springer-Verlag: Berlin, Heidelberg, New York, 1996.
- Metrohm AG. Ion-selective electrodes (ISE), Metrohm AG: Herisau, Switzerland, 2015. 8.109.8042EN
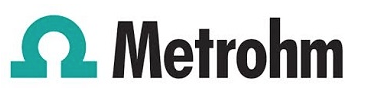
This information has been sourced, reviewed and adapted from materials provided by Metrohm AG.
For more information on this source, please visit Metrohm AG.