Sponsored by EDAXMar 2 2021
Also known as 3D printing, additive manufacturing (AM) is a disruptive new manufacturing technique that is employed to create metallic parts with enhanced mechanical properties in contrast to other traditional techniques.
3D printing can be performed on various metallic alloys with a range of processes, such as electron beam melting, direct metal laser melting and direct metal laser sintering (DMLS).
The microstructure of the material is influenced by each process, which in turn affects the mechanical characteristics. Electron backscatter diffraction (EBSD) is an effective technique for the characterization of microstructure and provides a more detailed understanding of the microstructure-property relationships.
Results and Discussion
EBSD is helpful to quantify several microstructural features, such as grain size, crystallographic orientation, phase distribution and grain boundary structure. Figure 1 presents an example from an AM 316-L stainless steel sample fabricated utilizing laser powder-bed fusion.
A combined EBSD image quality and inverse pole figure (IPF) map is presented in Figure 1a, where the color of each point correlates to their surface normal orientation. A combined EBSD image quality and grain map is depicted in Figure 1b, where points of equivalent orientation are grouped and colored at random to represent morphology.
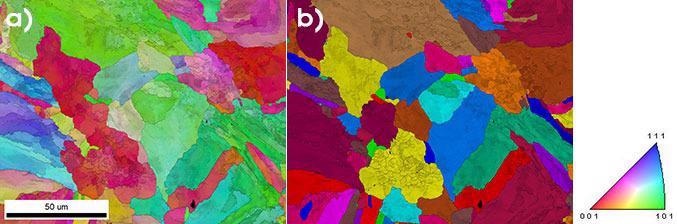
Figure 1. a) Inverse pole figure (IPF) orientation map and b) grain map from an additively manufactured 316-L stainless steel sample. Image Credit: EDAX
The Clarity™ EBSD Analysis System was used to collect this data. The next-generation direct electron detector removes the requirement for the phosphor screen and optics by directly imaging the diffracted electrons and excludes the distortions and blurring that are introduced by these conventional techniques.
Although this detector can acquire the conventional EBSD data presented in Figure 1, the high quality and sharp EBSD information acquired using the Clarity facilitates high fidelity EBSD measurements for optimized orientation precision. This functionality is critical because alloys made by L-PDB are prone to microstructures with high residual stresses as a result of fast solidification.
These AM steels are understood to have superior mechanical characteristics. Specifically, they provide improved ductility and strength compared to many wrought materials with equivalent compositions. This is caused by the dense dislocation network that is created after printing.
The features of this dislocation network differ with build parameters, along with locally inside a single build. This can be linked to various factors, such as cooling rates, local grain boundary characteristics, geometrical constraints and retained strains.
Statistically mapping out the dislocations as a function of build parameters and microstructure is a challenge when understanding the factors that affect these dislocation networks. X-ray methods do not have adequate spatial resolution and transmission electron microscopy cannot cover enough area.
Integrated with the performance of the Clarity Detector, high angular resolution EBSD (HR-EBSD) covers that divide by providing enough spatial resolution, collection automation and orientation precision to enable the quantified characterization of these microstructures.
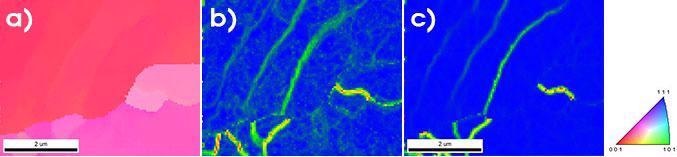
Figure 2. a) IPF orientation map and b) KAM map at higher spatial resolution. c) HR-KAM map calculated using cross-correlation HR-EBSD analysis, showing local defects within the microstructure. Image Credit: EDAX
Data gathered from a smaller field of view is presented in Figure 2 to demonstrate the microstructural information acquired with these techniques. The IPF orientation map of this field of view is depicted in Figure 2a.
The Kernel Average Misorientation (KAM) map determined from these quantified orientations is presented in Figure 2b. Figure 2c displays a high angular resolution KAM map, produced through the use of a cross correlation-based technique.
This HR-KAM map highlights the local defect structure inside the grains and suggests significantly decreased noise levels. This information is helpful to measure the geometrically-necessary dislocation density. These results are displayed in Figure 3.
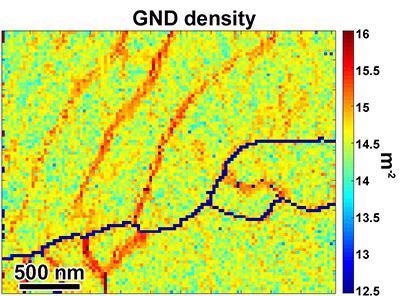
Figure 3. Geometrically necessary dislocation density map calculated using high fidelity EBSD patterns collected with the Clarity Detector and HR-EBSD analysis. Image Credit: EDAX
Further EBSD metrics are beneficial for the visualization of the cellular structures that emerge during rapid solidification and perform a crucial role in the advantageous properties that develop.
Figures 4a and 4b demonstrate an EBSD image quality map and a PRIAS™ (center) map from the same area, respectively. The dislocation cell structure is apparent in these images.
A combination of quantitative microstructure analysis via HR-EBSD and the defect information from these images provides a method of determining the structure-property relationships on a local scale in additively manufactured materials.
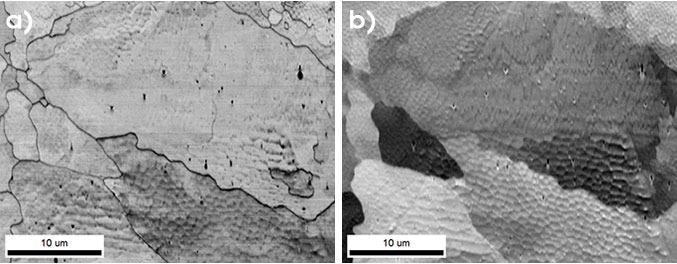
Figure 4. Visualization of a rapid solidification cellular microstructure developed during additive manufacturing using a) EBSD image quality and b) PRIAS center signals. Image Credit: EDAX
Conclusion
The stress state related to these dislocation networks is predicted to perform a crucial role in influencing mechanical properties. It still cannot be resolved with X-ray-based methods because of the spatial resolution.
An HR-EBSD approach is critical to better understand the local stress state in AM materials as a function of build parameters and microstructure and, in turn, allows the mechanical features of these materials to be determined.
The Clarity Detector delivers the high definition EBSD performance necessary to optimally capture the main characteristics included within these microstructures.
Acknowledgments
EDAX would like to thank Professor Josh Kacher of the Georgia Tech School of Materials Science and Engineering for assisting with the HR-EBSD analysis, giving valuable insight into the AM microstructures and for providing the sample.
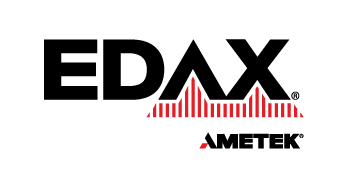
This information has been sourced, reviewed and adapted from materials provided by EDAX, LLC.
For more information on this source, please visit EDAX.